Review | Open Access
Potential mechanisms of intestinal microbes in bone metastasis of breast cancer
Nicky Gile1, Luke Johnston2
1Kentucky College of Osteopathic Medicine, University of Pikeville, 147 Sycamore Street, Pikeville, KY 41501, USA.
2Department of Statistics, University of Warwick, Coventry CV4 7AL, UK.
Correspondence: Nicky Gile (Kentucky College of Osteopathic Medicine, University of Pikeville, 147 Sycamore Street, Pikeville, KY 41501, USA; E-mail: N.Gile@hotmail.com).
Asia-Pacific Journal of Oncology 2025, 6: 37-46. https://doi.org/10.32948/ajo.2025.03.20
Received: 25 Dec 2024 | Accepted: 19 Mar 2025 | Published online: 31 Mar 2025
Key words breast cancer, bone metastasis, intestinal microbiota, immune regulation, metabolites
In recent years, research on the tumor microenvironment has become a key focus for understanding tumorigenesis, progression, and metastasis. The development of BM in BRCA involves complex biological processes, including interactions between tumor cells and the bone microenvironment, imbalances in osteoclast and osteoblast activity, and dysregulated immune responses [3]. Traditionally, BRCA cells are thought to promote osteoclast activation by secreting cytokines and chemokines, leading to increased bone resorption and facilitating cancer cell colonization in the bone niche. However, recent studies suggest that gut microbiota may play a crucial role in BRCA progression and BM by modulating the immune system and metabolic networks. This emerging area of research has garnered significant interest in cancer biology [4].
The gut microbiota is a vast and complex ecosystem residing within the host body and consists of diverse microbial communities, including bacteria, fungi, and viruses. Intestinal microbiota not only play a crucial role in the host’s nutritional metabolism but also influence the onset and progression of systemic diseases through immune regulation and inflammatory responses. Numerous studies have confirmed that dysbiosis is closely associated with various cancers, including colorectal cancer, liver cancer, pancreatic cancer, and BRCA [5, 6]. For example, research has shown that certain microbial communities, such as Escherichia coli, Clostridium, and Lactobacillus, contribute to cancer development by producing pro-inflammatory cytokines, toxins, or metabolites [7].
In BRCA, the intestinal microbiota is closely linked to estrogen biotransformation. The gut microbiota regulates the enterohepatic circulation of estrogen, influencing systemic estrogen levels and contributing to the development of hormone receptor-positive BRCA [8]. Additionally, patients with BRCA exhibit significant gut microbiota imbalances, characterized by a reduced number of probiotics, an increase in pathogenic bacteria, and markedly decreased microbial diversity and stability [9].
Bone metabolism is a dynamic equilibrium process regulated by osteoblasts and osteoclasts. Intestinal microbiota affects bone formation and resorption through its metabolites, such as short-chain fatty acids (SCFAs), and immune regulatory mechanisms, thereby maintaining bone homeostasis [10]. SCFAs, such as butyric acid and propionic acid, produced through microbial metabolism, regulate bone homeostasis by interacting with G-protein-coupled receptors (GPCRs), inhibiting osteoclast activity, and promoting osteoblast function [11].
Second, the gut microbiota can influence T-cell differentiation through immune regulation, particularly by modulating the balance between regulatory T cells (Tregs) and T helper 17 (Th17) cells. Notably, interleukin-17 (IL-17), secreted by Th17 cells, plays a crucial role in promoting bone resorption [12]. An imbalance in the gut microbiota can lead to systemic inflammation, compromised intestinal barrier function, and translocation of microbial components, such as lipopolysaccharides (LPS), into the bloodstream. This process activates inflammatory pathways, induces systemic inflammatory responses, and facilitates cancer cell growth in the bone microenvironment [13].
Although most existing research focuses on the relationship between gut microbiota and cancer, studies investigating how intestinal microbes influence BRCA bone metastasis are still in their infancy. However, indirect evidence suggests that the gut microbiota may impact the bone microenvironment through immune system modulation. For instance, IL-17-mediated osteoclast activation induced by the gut microbiota has been linked to the promotion of bone metastases [14]. Additionally, microbial metabolites, such as short-chain fatty acids (SCFAs) and bile acids, can enhance cancer cell proliferation and invasiveness by modulating host metabolism. Furthermore, gut microbiota imbalance contributes to chronic systemic inflammation, creating a favorable microenvironment for cancer cell metastasis to the bone [15, 16].
Therefore, further research on the mechanisms by which intestinal microbes influence BRCA bone metastasis is essential for understanding the systemic regulation of cancer metastasis. Moreover, these insights may pave the way for novel therapeutic strategies that target the gut microbiota.
Invasion and dissemination of tumor cells
The initial step in BRCA bone metastasis involves tumor cells acquiring invasive properties at the primary site, disrupting the thin layer of the extracellular matrix, and infiltrating the lymphatic and circulatory systems. This process is primarily driven by epithelial-mesenchymal transition (EMT), which enables BRCA cells to lose their epithelial polarity and gain motility and invasiveness [17].
Hashemi et al. emphasized the role of EMT in BRCA malignancy and drug resistance, highlighting that BRCA cells enhance their migratory ability through EMT induction. During this process, increased expression of N-cadherin and vimentin, coupled with reduced E-cadherin levels, facilitates EMT-driven BRCA metastasis [18]. Research indicates that Various regulatory factors and signaling pathways are involved in EMT modulation, including the TGF-β signaling pathway, Wnt/β-catenin signaling cascade, and Notch signaling mechanism [19]. For instance, a study by Chen et al. found that ICAM1 promotes bone metastasis in triple-negative BRCA patients via the integrin-mediated TGF-β/EMT signaling pathway, significantly affecting survival and quality of life [20]. Matrix metalloproteinases (MMPs) are a group of structurally and functionally related endopeptidases that play a crucial role in promoting tumor invasion and metastasis by degrading proteins in the extracellular matrix (ECM). Under normal conditions, their activities are tightly regulated to prevent tissue damage. However, in cancer, this regulation is often disrupted, allowing tumor cells to invade the surrounding tissues.
Different levels of MMP expression have been observed in patients with BRCA. Studies have shown that BRCA cells secrete MMPs, leading to ECM degradation and creating pathways for cell migration [21]. Chabottaux et al. found that MMPs are involved in multiple stages of cancer progression, including tumor growth, angiogenesis and metastasis. By breaking down ECM components, MMPs enhance tumor cell migration and aggressiveness [22].
Additionally, a study by Köhrmann et al. revealed that the regulation of MMP-8, MMP-10, MMP-12, and MMP-27 is associated with tumor grade. In comparison to G2 tissue samples, the MMPs exhibited higher expression levels in G3 samples. Furthermore, compared to healthy tissues, the expression of MMP-7 and MMP-27 mRNA is significantly lower in tumor samples [23].
Survival and migration in the circulation
Once cancerous cells enter the bloodstream, they form circulating tumor cells (CTCs). In the circulatory system, BRCA cells encounter significant challenges, including high shear stress from blood flow and immune surveillance mechanisms. To enhance their survival, these cells bind to platelets, forming protective microemboli that help them evade immune recognition and clearance [24].
For example, Anvari et al. found that CTCs rapidly bind to platelets upon entering the circulatory system. This interaction occurs through receptor-ligand binding, and platelet attachment facilitates CTC adhesion to the vascular wall, thereby promoting metastasis [25]. Research has shown that platelets mediate aggregation with cancer cells by interacting with P-selectin glycoprotein ligand-1 (PSGL-1) on the cancer cell surface. This interaction not only shields CTCs from immune attacks but also enhances their adhesion to endothelial cells [26]. Additionally, platelets cluster around tumor cells, forming a protective barrier that improves CTC survival and facilitates their attachment to distant organ endothelial cells [27]. Furthermore, cancer cells can stimulate platelet activation and induce the production of various growth factors, such as transforming growth factor-beta (TGF-β) and vascular endothelial growth factor (VEGF). These factors promote epithelial-mesenchymal transition (EMT) and angiogenesis, thereby increasing the aggressiveness and metastatic potential of tumor cells [28].
Immune evasion is a crucial factor in tumor migration, as CTCs express immunosuppressive molecules, such as programmed death-ligand 1 (PD-L1), to inhibit T-cell activity and evade immune surveillance [29]. A study by Darga et al. demonstrated that PD-L1 expression in CTCs is closely associated with BRCA prognosis. In patients with metastatic BRCA, higher PD-L1 expression in CTCs correlates with poorer overall survival and shorter progression-free survival. This finding suggests that CTCs promote tumor progression by suppressing T cell function through PD-L1 expression [30].
Additionally, studies have demonstrated that CTCs can inhibit T-cell activation and function by expressing PD-L1, which binds to PD-1 receptors on the surface of T cells, thereby enabling immune evasion. This mechanism plays a critical role in bone metastasis (BM) of BRCA [31]. Moreover, some CTCs exhibit the characteristics of cancer stem cells (CSCs), which enhances their survival, migration, and colonization abilities [32].
Colonization of neoplastic cells within the bone marrow microenvironment
BRCA cells colonize the bone marrow microenvironment through interactions with endothelial cell adhesion molecules (CAMs) and chemokines. These cells express adhesion molecules, such as integrins and selectins, which facilitate their attachment to and penetration of the bone marrow endothelial cells [33]. Zhong et al. demonstrated that E-selectin plays a crucial role in the adhesion and extravasation of cancer cells. E-selectin, an adhesion molecule expressed on endothelial cells, binds to specific ligands on the surface of cancer cells, mediating their rolling and adhesion to the vascular endothelium. This interaction promotes cancer cell extravasation and subsequent bone marrow metastasis [34].
Furthermore, Moritz et al. demonstrated that BRCA cells can influence their ability to colonize and metastasize in bone tissue by expressing specific integrin subunits, such as α2β1. In a mouse model, MDA-MB-231 cells overexpressing the α2 integrin subunit exhibited increased tumor dissemination and bone metastasis. However, this overexpression had minimal impact on tumor formation and bone destruction, suggesting that α2β1 integrin may play a biphasic role in BRCA bone metastasis [35]. In addition, chemokines secreted by the bone marrow microenvironment, such as CXCL12 (also known as stromal cell-derived factor 1, SDF-1), drive cancer cells toward the bone marrow by binding to the CXCR4 receptors on the surface of BRCA cells. This chemotactic mechanism promotes the colonization of cancer cells in the bone marrow [36]. Studies have shown that the CXCL12-CXCR4 interaction activates downstream signaling cascades, including the PI3K/AKT and MAPK/ERK pathways, which enhance cancer cell survival, proliferation, and invasion. This signaling network helps BRCA cells adapt to and thrive in the bone marrow microenvironment [37, 38].
Within the bone marrow microenvironment, some BRCA cells enter a dormant state and cease to proliferate. However, these dormant cells can be reactivated in response to specific stimuli from the bone microenvironment. Bone matrix factors, such as transforming growth factor-beta (TGF-β) and insulin-like growth factors (IGFs), promote the re-entry of dormant cells into the cell cycle, leading to the formation of metastatic foci [39].
Stimulation of osteoclasts and subsequent bone degradation
In the bone marrow microenvironment, BRCA cells secrete various factors that stimulate the maturation and activation of osteoclasts, leading to osteolysis. This process creates a vicious cycle of bone destruction and tumor progression, which is a hallmark of bone metastasis (BM) in BRCA. One key factor secreted by BRCA cells is parathyroid hormone-related protein (PTHrP), which directly activates osteoclasts and promotes bone degradation. Yin et al. showed that transforming growth factor-beta (TGF-β), stored in the bone matrix, is released during bone resorption, further stimulating the secretion of PTHrP by BRCA cells [40]. Additionally, the breakdown of the bone matrix releases stored growth factors, such as TGF-β and insulin-like growth factors (IGFs), which facilitate cancer cell proliferation and invasiveness. This cycle amplifies bone loss and tumor growth.
Blocking the TGF-β signaling pathway has been found to reduce PTHrP secretion in BRCA cells, thereby decreasing both BM progression and bone destruction. Research has shown that PTHrP expression is strongly associated with bone metastasis in patients with BRCA. In one study, the expression level of PTHrP was detected in over 90% of bone metastasis samples from patients with BRCA, whereas its expression rate in primary tumors was approximately 50%. This finding highlights the significant role of PTHrP in the onset and progression of bone metastasis in BRCA [41].
McCoy et al. found that interleukin-11 (IL-11) secreted by BRCA cells promotes osteoclast production by increasing the number of osteoclast precursors [42]. The development and activation of osteoclasts are primarily regulated by receptor activator of nuclear factor kappa B ligand (RANKL). However, Liang et al. demonstrated that IL-11 activates c-Myc through the JAK1/STAT3 signaling pathway, promoting osteoclastogenesis independently of RANKL and contributing to osteolysis in BRCA bone metastasis. Mechanistic studies have shown that IL-11 acts downstream of the JAK1/STAT3 signaling cascade, activating STAT3 and leading to the upregulation of c-Myc expression, which is an essential factor for osteoclast formation. These findings underscore the critical role of IL-11 and its molecular pathway in osteolysis mediated by BRCA bone metastasis [43].
There is also a strong interaction between tumor cells and bone marrow immune cells, particularly macrophages and T-cells. IL-17-mediated Th17 cell development is a key mechanism of osteoclast activation. BRCA cells further exacerbate bone destruction by modulating the immune microenvironment of the bone [44]. Zhu et al. found that BRCA-associated macrophages express IL-17, which directly enhances the invasiveness of BRCA cell lines, suggesting that IL-17 plays a significant role in BRCA progression [45]. Research indicates that IL-17, produced by Th17 cells, is crucial for BRCA invasion and metastasis. IL-17 facilitates tumor cell proliferation and migration by driving inflammatory responses and angiogenesis [44].
Tang et al. discovered that IL-17A regulates autophagy and promotes osteoclast differentiation via the ERK/mTOR/Baclin1 pathway. This study provides new insights into the role of IL-17 in bone destruction, further emphasizing its importance in BRCA bone metastasis [46].
Generation of metabolites
Short-chain fatty acids (SCFAs), such as propionic, acetic, and butyric acids, are produced by the gut microbiota through the fermentation of complex carbohydrates, such as dietary fiber. A study by Tao Zhou found that a higher dietary fiber intake is associated with an increased bone mineral density in the heel bone. Moreover, genetic variations in SCFA synthesis by the gut microbiota can influence this association, suggesting that SCFAs play a critical role in the impact of dietary fiber on bone health [47].
SCFAs affect bone metabolism through multiple pathways, including lowering intestinal pH, promoting mineral dissolution, and increasing calcium bioavailability, thereby improving calcium absorption in the body. Research has demonstrated that total SCFA concentration is negatively correlated with pH, following a decreasing trend from the cecum to the proximal colon, distal colon, and rectum. This suggests that SCFA production lowers intestinal pH, potentially influencing mineral solubility and absorption.
Hunt et al. found that SCFAs stimulate intestinal endocrine L cells to produce glucagon-like peptide 1 (GLP-1) and glucagon-like peptide 2 (GLP-2) by activating free fatty acid receptors (FFAR2 and FFAR3). GLP-2, in particular, is known to enhance the growth of intestinal epithelial cells, increase intestinal surface area, and consequently improve calcium absorption efficiency [48].
Additionally, SCFAs can reach bone tissue through the bloodstream and directly influence osteoblast activity, thereby regulating the balance between bone formation and resorption. Lucas et al. demonstrated that SCFAs act as in vivo regulators of bone mass and osteoclast metabolism. In a mouse model, treatment with SCFAs and a fiber-rich diet significantly increased bone mass, reduced inflammation, and prevented bone loss associated with menopause. This effect is primarily mediated by the inhibition of osteoclast differentiation and bone resorption [10].
Similarly, Behler Janbeck et al. found that SCFAs regulate bone mass through their receptors, GPR41 and GPR43. In a mouse model, mice lacking GPR41 and GPR43 exhibited increased bone mass compared to wild-type mice. In vitro experiments further demonstrated that mesenchymal stem cells from bone marrow treated with acetic acid showed increased expression of adipocyte marker genes, whereas osteogenic marker gene expression declined. This finding suggests that SCFAs may influence bone metabolism by promoting adipogenesis rather than osteogenic differentiation [49].
These studies provide insights into the mechanisms by which SCFAs regulate bone metabolism via their direct effects on bone cells. Additionally, SCFAs have been implicated in enhancing cancer cell metastasis by acting on the same metabolic pathways (Figure 1).
Immune system modification
Intestinal microbiota plays a crucial role in breast cancer (BRCA) progression by modulating the host immune system. Research indicates that an imbalance in gut flora can lead to immune dysfunction, thereby influencing the initiation and progression of BRCA [50]. Gut microbiota affects bone metastasis (BM) in BRCA by regulating the balance of T-cell subsets. Specifically, it influences the immune system of patients with BRCA by promoting neutrophil production, enhancing secretory IgA expression, and facilitating the growth and development of regulatory T cells (Tregs), ultimately affecting BRCA development and treatment outcomes [51].
Gut microbiota dysbiosis may alter the ratio of Tregs to helper T cells (Th17), thereby affecting osteoclast activity. IL-17 and other pro-inflammatory factors secreted by Th17 cells enhance osteoclast activation and differentiation, accelerating bone destruction and creating a favorable microenvironment for cancer cell colonization in the bone [52].
A study by Liu et al. using a mouse model demonstrated that eliminating the gut microbiota can reduce the severity of multiple myeloma and its associated bone disease. Their research showed that antibiotic treatment significantly altered the gut microbiota composition, decreased myeloma burden, and improved bone health. Additionally, a reduction in serum IL-17 levels and a decrease in circulating Th17 cell frequency in the spleen were observed, suggesting that the gut microbiota may influence osteoclast activity by regulating Th17 cells [53].
Similarly, Peng et al. explored the impact of gut microbiota balance on postmenopausal osteoporosis by regulating the Th17/Treg cell ratio. Their research highlighted the essential role of the gut microbiota and its metabolic byproducts in shaping immune cell development and function. This regulation of Th17/Treg equilibrium influences osteoclast activity and bone metabolism, further demonstrating the intricate connection between the gut microbiota and bone health [54].
Moreover, alterations in gut microbiota composition can influence the quantity and function of myeloid-derived suppressor cells (MDSCs). MDSCs have immunosuppressive properties and can inhibit the secretion of cytokines such as TGF-β and IL-10, thereby suppressing anti-tumor immune responses and promoting tumor growth and metastasis [55, 56].
A study by Ashkenazi Preiser found that in a mouse model of colitis-associated cancer, the interaction between MDSCs and the gut microbiome contributed to tumor progression. Antibiotic treatment, which induces gut microbiota dysbiosis, leads to a reduction in MDSC accumulation, decreased immune suppression, and significantly lowers the tumor burden. These findings suggest that gut microbiota modifications can regulate MDSC function, thereby affecting tumor initiation and progression [57].
Systemic inflammation and intestinal barrier
The gut microbiota composition plays a crucial role in regulating systemic inflammation. Dysbiosis of certain microbial communities can lead to an increase in pro-inflammatory cytokines, such as tumor necrosis factor-alpha (TNF-α) and interleukin-6 (IL-6), which promote osteoclast activation and contribute to bone loss. For example, Zhong et al. demonstrated that an imbalance in the gut microbiota elevates IL-6 levels in tumors and serum, triggering the NF-κB signaling cascade and thereby influencing tumor progression [58].
Conversely, a healthy gut microbiota can stimulate the release of anti-inflammatory cytokines, such as IL-10, which inhibit osteoclast activity and protect bone integrity. Furthermore, gut microbiota interact with the host immune system through the translocation of microorganisms and their byproducts. Under pathological conditions, disruption of the intestinal barrier and microbial imbalance facilitate bacterial translocation into the portal vein, liver, and other ectopic sites, leading to inflammatory responses that can contribute to tumor formation and progression [59].
The integrity of the intestinal mucosal barrier is largely dependent on the gut microbiota. A healthy gut microbiota helps maintain intestinal mucosal integrity by promoting mucus layer formation and enhancing the expression of tight junction proteins, thereby preventing harmful substances such as endotoxins from entering the bloodstream [60].
A study by Allam Ndoul et al. explored how the intestinal microbiome influences epithelial permeability by regulating tight junction protein expression. The research found that gut microbiota imbalance leads to a reduction in tight junction protein levels, weakening intestinal barrier function and allowing bacteria and their metabolic products to enter the circulatory system, triggering systemic inflammatory responses [61]. Additionally, previous studies have shown that intestinal epithelial cells regulate mucus layer expression and the production of antimicrobial molecules by responding to signals from both symbiotic and pathogenic bacteria in the intestinal lumen, as well as cytokines derived from lamina propria cells, thereby maintaining intestinal homeostasis [62].
Gut microbiota dysbiosis can compromise intestinal barrier integrity, increase intestinal permeability, and facilitate the entry of bacterial endotoxins, such as lipopolysaccharides (LPS), into the bloodstream. Once in circulation, LPS can induce systemic inflammation, promote osteoclast activation, and accelerate bone destruction [63], thereby creating a favorable environment for bone metastasis in BRCA.
Additionally, a study by Candelli M. investigated the interactions between lipopolysaccharides (LPS) and gut microbiota in inflammatory bowel disease. Research has shown that LPS activates Toll-like receptor 4 (TLR4), triggering intestinal inflammatory responses, disrupting intestinal barrier function, and leading to gut microbiota imbalance [64].
Moreover, multiple studies have reported that gut microbiota significantly influences cancer cell metastasis, exhibiting both promoting [65-67] and inhibitory effects [68-70] (Table 1).
In conclusion, the intestinal microbiota affects the bone metastasis (BM) process of BRCA through systemic inflammation and alterations in intestinal barrier function. A deeper investigation of these mechanisms is expected to introduce novel concepts and strategies for preventing and treating BRCA bone metastasis.
The impact of the endocrine system
The intestinal microbiota plays a crucial role in the development and progression of BRCA by influencing the host endocrine system, particularly the estrogen metabolism. Estrogen has a significant impact on bone metastasis (BM), and decreased estrogen levels are closely associated with osteoporosis.
Ervin et al. investigated the function of gut microbiota-derived β-glucuronidase in estrogen reactivation and its subsequent influence on target cell gene expression. The study revealed that β-glucuronidase, produced by the intestinal flora, increases the circulating levels of active estrogen by deconjugating estrogen glucuronide. This may contribute to the development and progression of BRCA [71]. Additionally, Hu S. reviewed the key role of gut microbiota β-glucuronidase in female estrogen metabolism, highlighting that the enzyme's activity affects estrogen metabolism and circulation levels, potentially influencing BRCA risk [72].
The intestine is also an important source of serotonin (5-HT), which plays a role in bone metabolism. Gut-derived 5-HT reduces bone formation by inhibiting osteoblast function. The gut microbiota regulates the synthesis of 5-HT, influencing bone metabolism. Ge X. explored the interaction between the gut microbiome and 5-HT, as well as its impact on gut motility. Their study indicated that gut microbiota-derived metabolites, including bile acids and short-chain fatty acids (SCFAs), can influence 5-HT production, which in turn directly or indirectly regulates intestinal peristalsis [73].
The role of intracellular bacteria
The traditional view has long held that most tissues and organs in the human body, except for the intestines, mouth, and skin, exist in a sterile state, including tumor tissues. However, recent studies have identified unique intracellular bacteria in BRCA tissue. These bacteria primarily reside in the cytoplasm of tumor cells, allowing them to evade host immune surveillance and clearance.
Intracellular bacteria that persist within the host cells are now recognized as key contributors to BRCA progression. Research has shown that breast tissue is not sterile, that gut microbiota can influence primary tumor growth, and that intracellular bacteria may play a crucial role in tumor metastasis. Fu A. et al. confirmed the presence of distinct intracellular bacteria in BRCA tissues and demonstrated their pivotal role in tumor metastasis and colonization [67]. Their findings suggest that during metastasis, intracellular bacteria enhance the resistance of circulating tumor cells (CTCs) to fluid shear stress by remodeling the actin cytoskeleton, thereby improving the survival of host tumor cells [67].
Further studies have revealed that intracellular bacteria facilitate cytoskeletal remodeling in tumor cells by activating the RhoA/ROCK signaling pathway. This modification enhances tumor cell resistance to fluid shear stress, thereby improving their survival in circulation and promoting metastasis and colonization of distant organs. Future research should focus on elucidating the specific mechanisms of intracellular bacteria in BRCA and exploring how microbial insights can be leveraged to develop novel therapeutic strategies.
Table 1. Association of gut microorganisms with breast cancer metastasis. |
|||
Microorganisms involved |
Function |
Impact on migration |
References |
Clostridium difficile |
Bacterial colonization inhibits the accumulation of tumor-infiltrating T cells and NK cells. |
Promote migration |
[65] |
Clostridiaceae, Clostridium faecalis, Ruminococcus |
Microorganisms that produce β-glucuronidase convert the bound inactive form of estrogen to the de-bound biologically active form. |
Promote migration |
[66] |
Staphylococcus xylosus, Lactobacillus animalis, and Streptococcus cuniculi |
Enhanced resistance to FSS by recombinant actin cytoskeleton. |
Promote migration |
[67] |
E. coli |
Indole-propionic acid decreases the expression of waveform protein, FGFBP1, Snail, and β-catenin; and up-regulates the expression of E-calmodulin to inhibit epithelial-mesenchymal transition. |
Inhibition of cancer metastasis |
[68] |
E. coli |
Cadaverine reduces the motility and metastatic properties of cancer stem cells by restoring epithelial-mesenchymal transition. |
Inhibition of cancer metastasis |
[69] |
Lactobacillus, Bifidobacterium, E. coli |
Regulation of inflammation and immune response. |
Inhibition of cancer metastasis |
[70] |
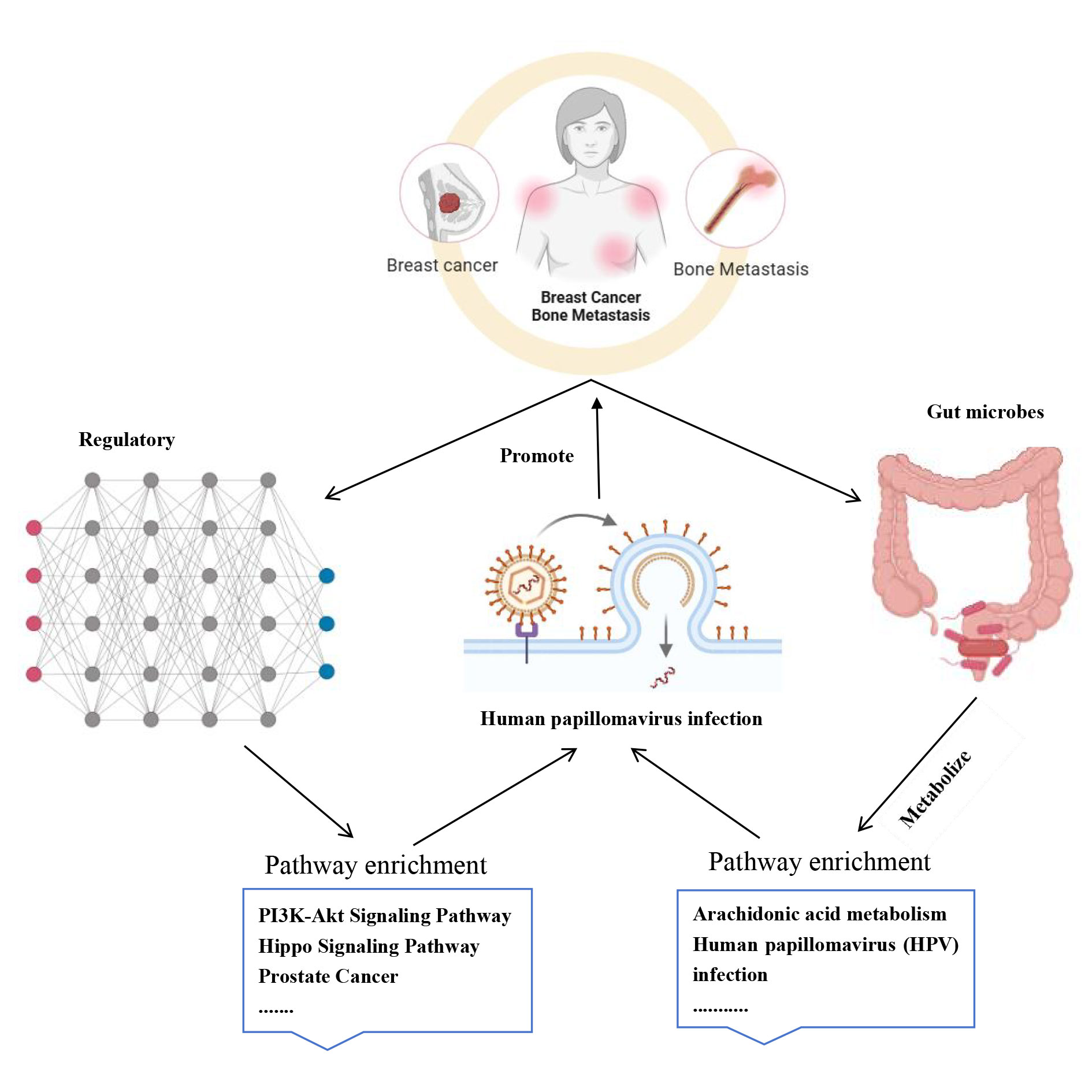
On one hand, gut microbiota influences bone homeostasis by modulating metabolites, such as short-chain fatty acids (SCFAs). SCFAs can directly act on osteoclasts and osteoblasts within bone tissue while maintaining the bone metabolism balance by promoting calcium absorption and inhibiting osteoclast activity. For instance, butyric and propionic acids have been shown to significantly inhibit osteoclast differentiation and activation by engaging G-protein-coupled receptors (GPCRs), thereby blocking the colonization and spread of BRCA cells in the bone [5].
Conversely, gut microbiota dysbiosis contributes to systemic inflammation, further exacerbating the vicious cycle of bone loss. Impairment of the intestinal barrier function allows endotoxins, such as lipopolysaccharides (LPS), to enter the bloodstream, subsequently activating the NF-κB signaling pathway and inducing the production of pro-inflammatory cytokines (e.g., IL-6 and TNF-α). These cytokines stimulate osteoclast activity, ultimately facilitating bone degradation and promoting metastasis.
BM in BRCA depends on immune evasion within the tumor microenvironment, and intestinal microorganisms play crucial roles in regulating the host immune system. This review discusses how the gut microbiota can indirectly influence bone metastasis by modulating the balance of T-cell subsets, particularly regulatory T cells (Tregs) and T-helper 17 (Th17) cells [6]. Research has shown that IL-17, secreted by Th17 cells, plays a key role in bone resorption, and its increased levels are associated with BM in BRCA. Additionally, a decrease in Tregs not only enhances osteoclast activity but also creates favorable conditions for the colonization of BRCA cells in the bone.
The impact of intestinal microbial metabolites on BM in BRCA is bidirectional. Conversely, SCFAs, such as propionic acid and butyric acid, exhibit anti-metastatic effects by reducing inflammation and regulating bone metabolism. Conversely, certain microbial metabolites (such as bile acids) may promote BM in BRCA by activating cancer cell migration and invasion pathways. This complexity underscores the need for a comprehensive consideration of both pro-tumorigenic and anti-cancer effects when developing therapeutic strategies targeting microbial metabolites.
Although the specific mechanisms of intestinal microbes in BRCA bone metastasis require further investigation, existing evidence suggests that modulating the gut microbiota could be a promising strategy for BM prevention and management. For instance, probiotics and prebiotics can reshape the gut microbiota composition, enhance immune homeostasis, and inhibit cancer cell colonization in the bone. Additionally, therapeutic approaches targeting microbial metabolites, such as short-chain fatty acids (SCFAs), have shown promising potential. However, it is crucial to explore how these strategies can be effectively integrated into immunotherapy (Figure 2).
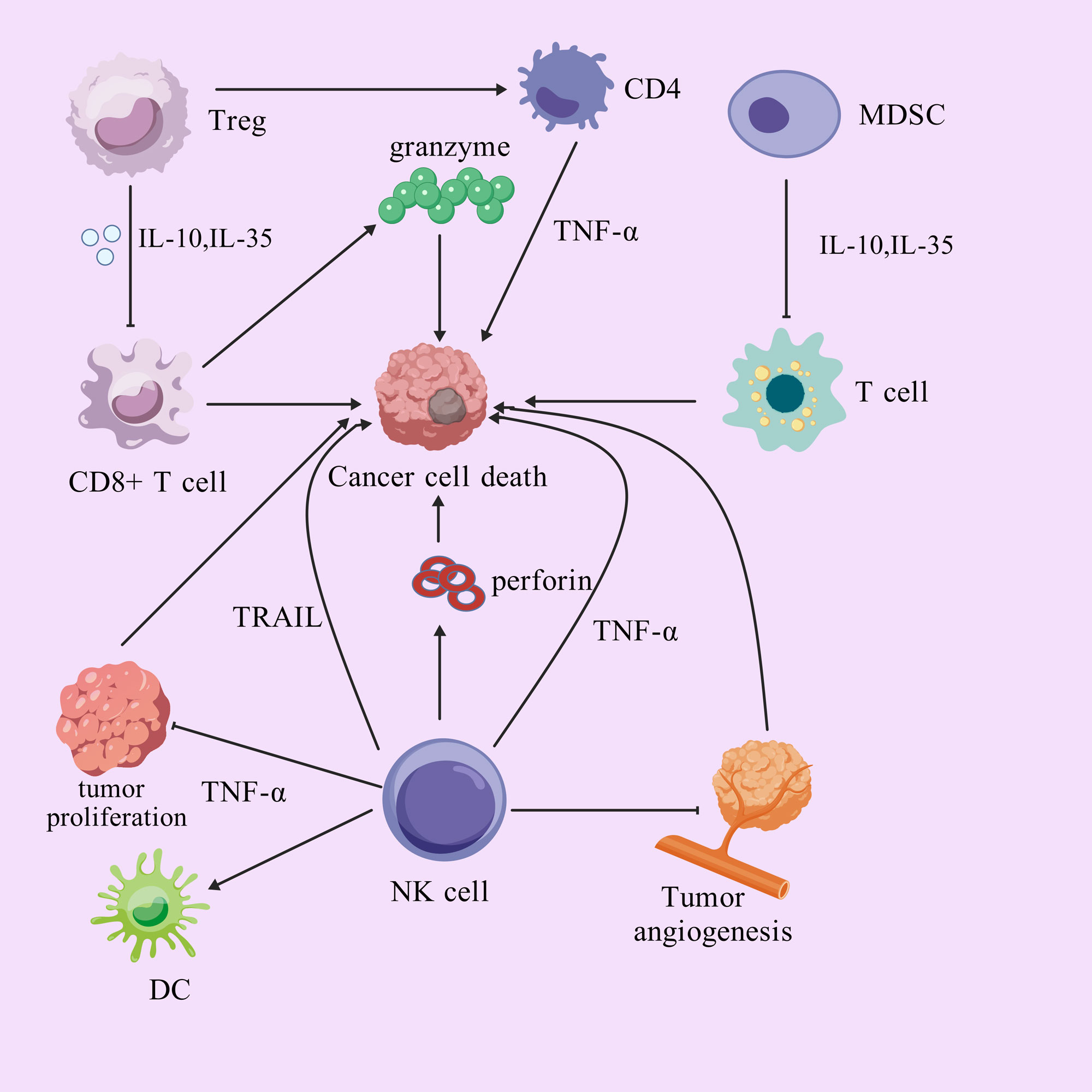
No applicable.
Ethics approval
No applicable.
Data availability
This narrative review is based on previously published studies and publicly available data. No new datasets were generated or analyzed for the current review.
Funding
None.
Authors’ contribution
Nicky Gile and Luke Johnston contributed to the conception, design, writing of this review article and figures drawing. Nicky Gile revised the review and submitted the final version of the manuscript.
Competing interests
The authors declare no competing interests.
- Bray F, Laversanne M, Sung H, Ferlay J, Siegel RL, Soerjomataram I, Jemal A: Global cancer statistics 2022: GLOBOCAN estimates of incidence and mortality worldwide for 36 cancers in 185 countries. CA Cancer J Clin 2024, 74(3): 229-263.
- Coleman RE: Metastatic bone disease: clinical features, pathophysiology and treatment strategies. Cancer Treat Rev 2001, 27(3): 165-176.
- Guise TA, Mohammad KS, Clines G, Stebbins EG, Wong DH, Higgins LS, Vessella R, Corey E, Padalecki S, Suva L, Chirgwin JM: Basic mechanisms responsible for osteolytic and osteoblastic bone metastases. Clin Cancer Res 2006, 12(20 Pt 2): 6213s-6216s.
- Garrett WS: Cancer and the microbiota. Science 2015, 348(6230): 80-86.
- Chen J, Pitmon E, Wang K: Microbiome, inflammation and colorectal cancer. Semin Immunol 2017, 32: 43-53.
- Routy B, Le Chatelier E, Derosa L, Duong CPM, Alou MT, Daillère R, Fluckiger A, Messaoudene M, Rauber C, Roberti MP et al: Gut microbiome influences efficacy of PD-1-based immunotherapy against epithelial tumors. Science 2018, 359(6371): 91-97.
- Parida S, Sharma D: The Microbiome-Estrogen Connection and Breast Cancer Risk. Cells 2019, 8(12): 1642.
- Kwa M, Plottel CS, Blaser MJ, Adams S: The Intestinal Microbiome and Estrogen Receptor-Positive Female Breast Cancer. J Natl Cancer Inst 2016, 108(8): djw029.
- Legesse Bedada T, Feto TK, Awoke KS, Garedew AD, Yifat FT, Birri DJ: Probiotics for cancer alternative prevention and treatment. Biomed Pharmacother 2020, 129: 110409.
- Lucas S, Omata Y, Hofmann J, Böttcher M, Iljazovic A, Sarter K, Albrecht O, Schulz O, Krishnacoumar B, Krönke G et al: Short-chain fatty acids regulate systemic bone mass and protect from pathological bone loss. Nat Commun 2018, 9(1): 55.
- McCabe LR, Irwin R, Schaefer L, Britton RA: Probiotic use decreases intestinal inflammation and increases bone density in healthy male but not female mice. J Cell Physiol 2013, 228(8): 1793-1798.
- Omenetti S, Pizarro TT: The Treg/Th17 Axis: A Dynamic Balance Regulated by the Gut Microbiome. Front Immunol 2015, 6: 639.
- Zhao M, Chu J, Feng S, Guo C, Xue B, He K, Li L: Immunological mechanisms of inflammatory diseases caused by gut microbiota dysbiosis: A review. Biomed Pharmacother 2023, 164: 114985.
- Chen Y, Wang X, Zhang C, Liu Z, Li C, Ren Z: Gut Microbiota and Bone Diseases: A Growing Partnership. Front Microbiol 2022, 13: 877776.
- Liu J, Tian R, Sun C, Guo Y, Dong L, Li Y, Song X: Microbial metabolites are involved in tumorigenesis and development by regulating immune responses. Front Immunol 2023, 14: 1290414.
- Zeng H, Umar S, Rust B, Lazarova D, Bordonaro M: Secondary Bile Acids and Short Chain Fatty Acids in the Colon: A Focus on Colonic Microbiome, Cell Proliferation, Inflammation, and Cancer. Int J Mol Sci 2019, 20(5): 1214
- Scimeca M, Trivigno D, Bonfiglio R, Ciuffa S, Urbano N, Schillaci O, Bonanno E: Breast cancer metastasis to bone: From epithelial to mesenchymal transition to breast osteoblast-like cells. Semin Cancer Biol 2021, 72: 155-164.
- Hashemi M, Arani HZ, Orouei S, Fallah S, Ghorbani A, Khaledabadi M, Kakavand A, Tavakolpournegari A, Saebfar H, Heidari H et al: EMT mechanism in breast cancer metastasis and drug resistance: Revisiting molecular interactions and biological functions. Biomed Pharmacother 2022, 155: 113774.
- Nieto MA, Huang RY, Jackson RA, Thiery JP: EMT: 2016. Cell 2016, 166(1): 21-45.
- Chen M, Wu C, Fu Z, Liu S: ICAM1 promotes bone metastasis via integrin-mediated TGF-β/EMT signaling in triple-negative breast cancer. Cancer Sci 2022, 113(11): 3751-3765.
- Bonnomet A, Brysse A, Tachsidis A, Waltham M, Thompson EW, Polette M, Gilles C: Epithelial-to-mesenchymal transitions and circulating tumor cells. J Mammary Gland Biol Neoplasia 2010, 15(2): 261-273.
- Chabottaux V, Noel A: Breast cancer progression: insights into multifaceted matrix metalloproteinases. Clin Exp Metastasis 2007, 24(8): 647-656.
- Köhrmann A, Kammerer U, Kapp M, Dietl J, Anacker J: Expression of matrix metalloproteinases (MMPs) in primary human breast cancer and breast cancer cell lines: New findings and review of the literature. BMC Cancer 2009, 9(1): 188.
- Labelle M, Begum S, Hynes RO: Platelets guide the formation of early metastatic niches. Proc Natl Acad Sci U S A 2014, 111(30): E3053-3061.
- Anvari S, Osei E, Maftoon N: Interactions of platelets with circulating tumor cells contribute to cancer metastasis. Sci Rep 2021, 11(1): 15477.
- Xue J, Deng J, Qin H, Yan S, Zhao Z, Qin L, Liu J, Wang H: The interaction of platelet-related factors with tumor cells promotes tumor metastasis. J Transl Med 2024, 22(1): 371.
- Fidler IJ: The pathogenesis of cancer metastasis: the 'seed and soil' hypothesis revisited. Nat Rev Cancer 2003, 3(6): 453-458.
- Zhou L, Zhang Z, Tian Y, Li Z, Liu Z, Zhu S: The critical role of platelet in cancer progression and metastasis. Eur J Med Res 2023, 28(1): 385.
- Steeg PS: Targeting metastasis. Nat Rev Cancer 2016, 16(4): 201-218.
- Darga EP, Dolce EM, Fang F, Kidwell KM, Gersch CL, Kregel S, Thomas DG, Gill A, Brown ME, Gross S et al: PD-L1 expression on circulating tumor cells and platelets in patients with metastatic breast cancer. PLoS One 2021, 16(11): e0260124.
- Jin M, Fang J, Peng J, Wang X, Xing P, Jia K, Hu J, Wang D, Ding Y, Wang X et al: PD-1/PD-L1 immune checkpoint blockade in breast cancer: research insights and sensitization strategies. Mol Cancer 2024, 23(1): 266.
- Pantel K, Alix-Panabières C: Liquid biopsy and minimal residual disease - latest advances and implications for cure. Nat Rev Clin Oncol 2019, 16(7): 409-424.
- Müller A, Homey B, Soto H, Ge N, Catron D, Buchanan ME, McClanahan T, Murphy E, Yuan W, Wagner SN et al: Involvement of chemokine receptors in breast cancer metastasis. Nature 2001, 410(6824): 50-56.
- Zhong L, Simoneau B, Tremblay P-L, Gout S, Simard MJ, Huot J: E-Selectin-Mediated Adhesion and Extravasation in Cancer. Encyclopedia of Cancer. 2017: 1618-1624.
- Moritz MNO, Merkel AR, Feldman EG, Selistre-de-Araujo HS, Rhoades Sterling JA: Biphasic α2β1 Integrin Expression in Breast Cancer Metastasis to Bone. Int J Mol Sci 2021, 22(13): 6906.
- Weilbaecher KN, Guise TA, McCauley LK: Cancer to bone: a fatal attraction. Nat Rev Cancer 2011, 11(6): 411-425.
- Wang J, Loberg R, Taichman RS: The pivotal role of CXCL12 (SDF-1)/CXCR4 axis in bone metastasis. Cancer Metastasis Rev 2006, 25(4): 573-587.
- Shi Y, Riese DJ, 2nd, Shen J: The Role of the CXCL12/CXCR4/CXCR7 Chemokine Axis in Cancer. Front Pharmacol 2020, 11: 574667.
- Kang Y, Siegel PM, Shu W, Drobnjak M, Kakonen SM, Cordón-Cardo C, Guise TA, Massagué J: A multigenic program mediating breast cancer metastasis to bone. Cancer Cell 2003, 3(6): 537-549.
- Yin JJ, Selander K, Chirgwin JM, Dallas M, Grubbs BG, Wieser R, Massagué J, Mundy GR, Guise TA: TGF-beta signaling blockade inhibits PTHrP secretion by breast cancer cells and bone metastases development. J Clin Invest 1999, 103(2): 197-206.
- Chen Y-C, Sosnoski DM, Mastro AM: Breast cancer metastasis to the bone: mechanisms of bone loss. Breast Cancer Res 2010, 12(6): 215.
- McCoy EM, Hong H, Pruitt HC, Feng X: IL-11 produced by breast cancer cells augments osteoclastogenesis by sustaining the pool of osteoclast progenitor cells. BMC Cancer 2013, 13(1): 16.
- Liang M, Ma Q, Ding N, Luo F, Bai Y, Kang F, Gong X, Dong R, Dai J, Dai Q et al: IL-11 is essential in promoting osteolysis in breast cancer bone metastasis via RANKL-independent activation of osteoclastogenesis. Cell Death Dis 2019, 10(5): 353.
- Shibabaw T, Teferi B, Ayelign B: The role of Th-17 cells and IL-17 in the metastatic spread of breast cancer: As a means of prognosis and therapeutic target. Front Immunol 2023, 14: 1094823.
- Zhu X, Mulcahy LA, Mohammed RAA, Lee AHS, Franks HA, Kilpatrick L, Yilmazer A, Paish EC, Ellis IO, Patel PM, Jackson AM: IL-17 expression by breast-cancer-associated macrophages: IL-17 promotes invasiveness of breast cancer cell lines. Breast Cancer Res 2008, 10(6): R95.
- Tang H, Zhu S, Chen K, Yuan S, Hu J, Wang H: IL-17A regulates autophagy and promotes osteoclast differentiation through the ERK/mTOR/Beclin1 pathway. PLoS One 2023, 18(2): e0281845.
- Zhou T, Wang M, Ma H, Li X, Heianza Y, Qi L: Dietary Fiber, Genetic Variations of Gut Microbiota-derived Short-chain Fatty Acids, and Bone Health in UK Biobank. J Clin Endocrinol Metab 2020, 106(1): 201-210.
- Hunt JE, Christiansen CB, Yassin M, Hartmann B, Offermanns S, Dragsted LO, Holst JJ, Kissow H: The Severity of DSS-Induced Colitis Is Independent of the SCFA-FFAR2/3-GLP-1 Pathway Despite SCFAs Inducing GLP-1 Secretion via FFAR2/3. Metabolites 2024, 14(7): 395.
- Behler-Janbeck F, Baranowsky A, Yorgan TA, Jaeckstein MY, Worthmann A, Fuh MM, Gunasekaran K, Tiegs G, Amling M, Schinke T, Heeren J: The short-chain fatty acid receptors Gpr41/43 regulate bone mass by promoting adipogenic differentiation of mesenchymal stem cells. Front Endocrinol (Lausanne) 2024, 15: 1392418.
- Wenhui Y, Zhongyu X, Kai C, Zhaopeng C, Jinteng L, Mengjun M, Zepeng S, Yunshu C, Peng W, Yanfeng W, Huiyong S: Variations in the Gut Microbiota in Breast Cancer Occurrence and Bone Metastasis. Front Microbiol 2022, 13: 894283.
- Xue K, Li J, Huang R: The immunoregulatory role of gut microbiota in the incidence, progression, and therapy of breast cancer. Front Cell Infect Microbiol 2024, 14: 1411249.
- Contino KF, Yadav H, Shiozawa Y: The gut microbiota can be a potential regulator and treatment target of bone metastasis. Biochem Pharmacol 2022, 197: 114916.
- Liu J, Xie F, Yi Z-g, Ma T, Tie W-t, Li Y-h, Bai J, Zhang L-s: Gut microbiota deficiency ameliorates multiple myeloma and myeloma-related bone disease by Th17 cells in mice models. J Cancer 2023, 14(17): 3191-3202.
- Qi P, Xie R, Liu H, Zhang Z, Cheng Y, Ma J, Wan K, Xie X: Mechanisms of gut homeostasis regulating Th17/Treg cell balance in PMOP. Front Immunol 2024, 15: 1497311.
- Liu X, Chen Y, Zhang S, Dong L: Gut microbiota-mediated immunomodulation in tumor. J Exp Clin Cancer Res 2021, 40(1): 221.
- Feng P, Xue X, Bukhari I, Qiu C, Li Y, Zheng P, Mi Y: Gut microbiota and its therapeutic implications in tumor microenvironment interactions. Front Microbiol 2024, 15: 1287077.
- Ashkenazi-Preiser H, Reuven O, Uzan-Yulzari A, Komisarov S, Cirkin R, Turjeman S, Even C, Twaik N, Ben-Meir K, Mikula I, Jr et al: The Cross-talk Between Intestinal Microbiota and MDSCs Fuels Colitis-associated Cancer Development. Cancer Res Commun 2024, 4(4): 1063-1081.
- Zhong W, Wu K, Long Z, Zhou X, Zhong C, Wang S, Lai H, Guo Y, Lv D, Lu J, Mao X: Gut dysbiosis promotes prostate cancer progression and docetaxel resistance via activating NF-κB-IL6-STAT3 axis. Microbiome 2022, 10(1): 94.
- Singh A, Nayak N, Rathi P, Verma D, Sharma R, Chaudhary A, Agarwal A, Tripathi YB, Garg N: Microbiome and host crosstalk: A new paradigm to cancer therapy. Semin Cancer Biol 2021, 70 71-84.
- Dmytriv TR, Storey KB, Lushchak VI: Intestinal barrier permeability: the influence of gut microbiota, nutrition, and exercise. Front Physiol 2024, 15: 1380713.
- Allam-Ndoul B, Castonguay-Paradis S, Veilleux A: Gut Microbiota and Intestinal Trans-Epithelial Permeability. Int J Mol Sci 2020, 21(17) 6402.
- Okumura R, Takeda K: The role of the mucosal barrier system in maintaining gut symbiosis to prevent intestinal inflammation. Semin Immunopathol 2024, 47(1): 2.
- Li Q, von Ehrlich-Treuenstätt V, Schardey J, Wirth U, Zimmermann P, Andrassy J, Bazhin AV, Werner J, Kühn F: Gut Barrier Dysfunction and Bacterial Lipopolysaccharides in Colorectal Cancer. J Gastrointest Surg 2023, 27(7): 1466-1472.
- Candelli M, Franza L, Pignataro G, Ojetti V, Covino M, Piccioni A, Gasbarrini A, Franceschi F: Interaction between Lipopolysaccharide and Gut Microbiota in Inflammatory Bowel Diseases. Int J Mol Sci 2021, 22(12): 6242.
- Parhi L, Alon-Maimon T, Sol A, Nejman D, Shhadeh A, Fainsod-Levi T, Yajuk O, Isaacson B, Abed J, Maalouf N et al: Breast cancer colonization by Fusobacterium nucleatum accelerates tumor growth and metastatic progression. Nat Commun 2020, 11(1): 3259.
- Yang J, Tan Q, Fu Q, Zhou Y, Hu Y, Tang S, Zhou Y, Zhang J, Qiu J, Lv Q: Gastrointestinal microbiome and breast cancer: correlations, mechanisms and potential clinical implications. Breast Cancer 2017, 24(2): 220-228.
- Fu A, Yao B, Dong T, Chen Y, Yao J, Liu Y, Li H, Bai H, Liu X, Zhang Y et al: Tumor-resident intracellular microbiota promotes metastatic colonization in breast cancer. Cell 2022, 185(8): 1356-1372.
- Sári Z, Mikó E, Kovács T, Jankó L, Csonka T, Lente G, Sebő É, Tóth J, Tóth D, Árkosy P et al: Indolepropionic Acid, a Metabolite of the Microbiome, Has Cytostatic Properties in Breast Cancer by Activating AHR and PXR Receptors and Inducing Oxidative Stress. Cancers (Basel) 2020, 12(9): 2411.
- Kovács T, Mikó E, Vida A, Sebő É, Toth J, Csonka T, Boratkó A, Ujlaki G, Lente G, Kovács P et al: Cadaverine, a metabolite of the microbiome, reduces breast cancer aggressiveness through trace amino acid receptors. Sci Rep 2019, 9(1): 1300.
- Jeraldo P, Hernandez A, Nielsen HB, Chen X, White BA, Goldenfeld N, Nelson H, Alhquist D, Boardman L, Chia N: Capturing One of the Human Gut Microbiome's Most Wanted: Reconstructing the Genome of a Novel Butyrate-Producing, Clostridial Scavenger from Metagenomic Sequence Data. Front Microbiol 2016, 7: 783.
- Ervin SM, Li H, Lim L, Roberts LR, Liang X, Mani S, Redinbo MR: Gut microbial β-glucuronidases reactivate estrogens as components of the estrobolome that reactivate estrogens. J Biol Chem 2019, 294(49): 18586-18599.
- Hu S, Ding Q, Zhang W, Kang M, Ma J, Zhao L: Gut microbial beta-glucuronidase: a vital regulator in female estrogen metabolism. Gut Microbes 2023, 15(1): 2236749.
- Ge X, Pan J, Liu Y, Wang H, Zhou W, Wang X: Intestinal Crosstalk between Microbiota and Serotonin and its Impact on Gut Motility. Curr Pharm Biotechnol 2018, 19(3): 190-195.
Asia-Pacific Journal of Oncology
print ISSN: 2708-7980, online ISSN: 2708-7999
Copyright © Asia Pac J Oncol. This work is licensed under a Creative Commons Attribution-NonCommercial-No Derivatives 4.0 International (CC BY-NC-ND 4.0) License.