Review | Open Access
Innovative and future approaches for ovarian cancer detection and evaluation: a comprehensive review
Fomukong Tasinda Raphael1, Simon Nabirye2
1Sinda Foundation Clinic Bonamoussadi, Douala, Cameroon.
2School of Health Sciences, Kampala University, Ggaba, Kampala, Uganda.
Correspondence: Simon Nabirye (School of Health Sciences, Kampala University, Ggaba, Kampala, Uganda; E-mail: S.Nabirye@hotmail.com).
Asia-Pacific Journal of Oncology 2025, 6: 18-26. https://doi.org/10.32948/ajo.2025.01.20
Received: 13 Sep 2024 | Accepted: 22 Jan 2025 | Published online: 04 Feb 2025
Key words ovarian cancer, CA125, biomarkers, pathways, autophagy
In 2022, epidemiological projections from the American Cancer Society indicated that 19,800 individuals in the United States will receive a new diagnosis of OC, while the disease is anticipated to claim the lives of 12,810 women [10]. The elevated mortality associated with high-grade serous carcinoma (HGSC) is predominantly attributable to challenges in disease detection, particularly during the early stages when clinical interventions demonstrate optimal efficacy [11]. Furthermore, chemotherapeutic interventions for OC substantially compromise patients' quality of life owing to severe adverse reactions, including fatigue, arthralgia, and neurotoxicity [12, 13]. Hence, advances in more precise and efficacious techniques for detecting OC in its incipient stages could yield substantial improvements in patient prognosis.
Pharmaceutical delivery and co-delivery mechanisms within ovarian carcinoma management represent critical avenues for therapeutic interventions. Drug delivery systems have been engineered to incorporate either single-target compounds or multiple-target agents, thereby enhancing the efficiency of drug release and mitigating the associated toxicity. This study aimed to assess the current understanding of OC encompassing its related signalling pathways, targeted treatment approaches, and innovative drug delivery methodologies.
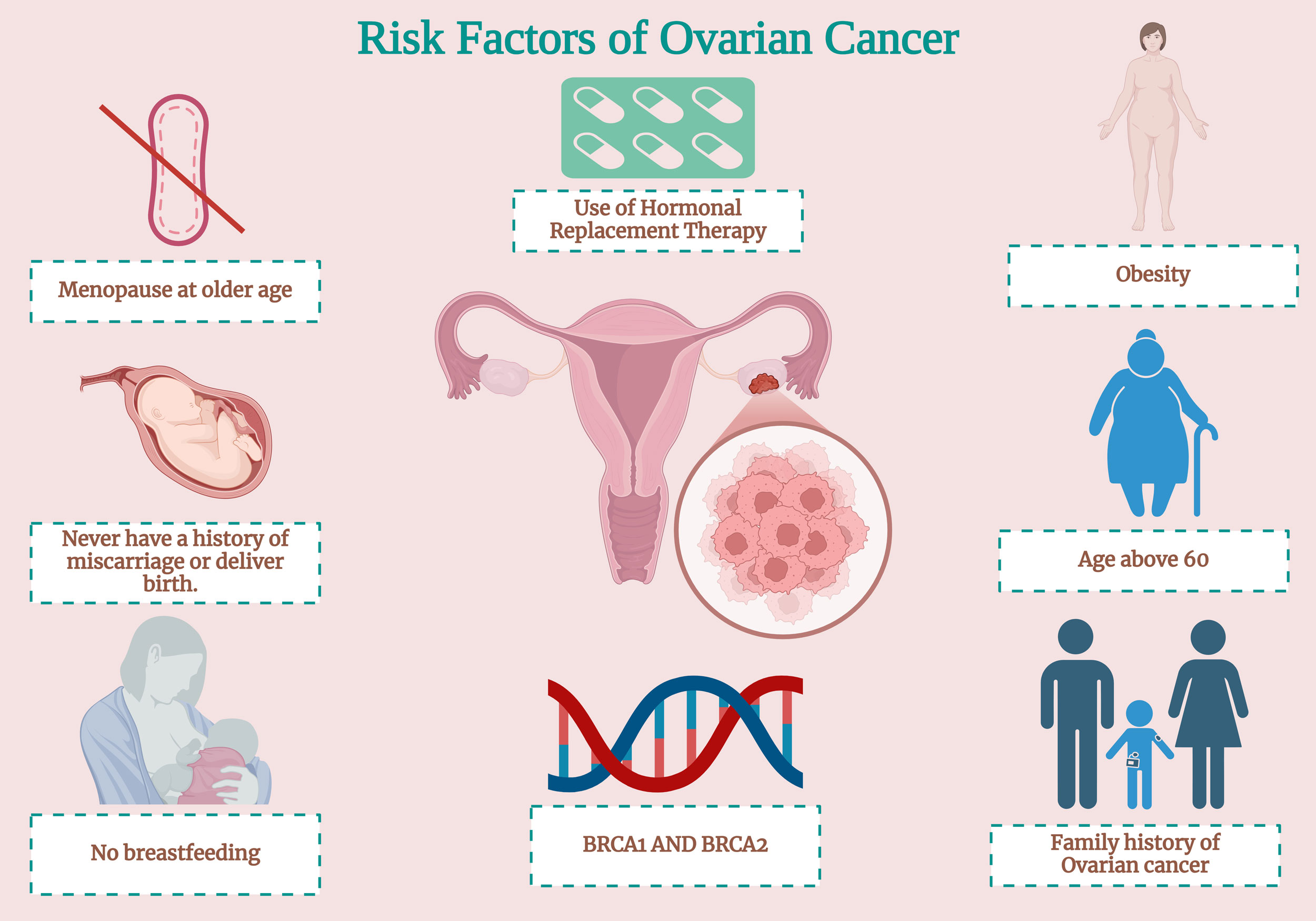
Ultrasound screening technology facilitates the in-depth examination of ovarian structures, enabling the identification of potential morphological alterations that may indicate the emergence of malignant neoplasms [24]. Transvaginal ultrasonography (TVU) is the predominant imaging method used for the detection of OC. This enables healthcare practitioners to discern anomalies in ovarian tissue morphology and dimension. Using TVU, radiologists conduct assessments of specific clinical attributes according to the International Ovarian Tumor Analysis (IOTA) criteria. The evaluation process involved scrutinizing various features, including papillary projections, ascites, and patterns of internal blood flow. These observations serve as crucial indicators for estimating the probability and extent of malignant masses [25].
CA125
CA125, a glycoprotein expressed on the surface of epithelial cells, is the predominant biomarker for OC and facilitates the proliferation and dissemination of malignant cells [26]. Preliminary studies on CA125 revealed that approximately 80% of individuals with OC exhibited elevated serum levels of this protein, exceeding 35 U/mL [27]. Unfortunately, further investigations into the clinical benefit of CA125 screening have yet to demonstrate substantial benefits to patients, primarily due to a lack of clinical sensitivity for CA125 at the early stages of the disease. The efficacy of CA125 levels in enhancing the differentiation of ovarian masses appears to be constrained when integrated into multifaceted diagnostic strategies during preoperative evaluation [28].
In 2016, a landmark study on OC screening was published by Jacobs et al., who presented the results of the United Kingdom Collaborative Trial of Ovarian Cancer Screening (UKCTOCS). This trial is one of the most extensive randomized controlled trials in OC research. This study examined the impact of early screening using the Risk of Ovarian Cancer Algorithm (ROCA) on OC mortality in over 200,000 post-menopausal women over an average of 11.1 years. To stratify patient risk, ROCA considers baseline and serially collected CA125 levels, patient age, menopausal status, and other risk variables, such as BRCA status. Although the study revealed better survival in the screened groups, the results were insignificant, emphasizing CA125's limitations [29]. Although CA125 exhibits suboptimal efficacy as a screening biomarker, it yields substantial clinical advantages when used as a postoperative parameter. This marker is particularly valuable for assessing therapeutic responses and facilitating the detection of disease recurrence in patients. Table 1 describes the therapeutic value of newly discovered biomarkers in treating OC and their possible future development.
Table 1. OC treatment with new biomarkers and its possible advancement. |
||||
Newly identified biomarkers |
Origin |
Prospective therapeutic function |
Mechanism |
References |
Cu isotope ratio δ65Cu |
Serum |
The quantification of this substance in blood plasma or serum presents a potential avenue for non-invasive diagnostic or prognostic biomarker applications. |
The upregulation of specific proteins, including ceruloplasmin, CTR1 (copper transporter 1), and ATP7A/B , in cellular processes may exert an influence on the isotopic composition. |
[30] |
Extracellular Vesicles (EVs) |
Blood, Ascitic fluid, urine etc. |
EVs demonstrate potential as delivery vehicles for various therapeutic agents, including chemotherapeutic drugs, siRNAs, and CRISPR/Cas9 components, with specific application in targeting ovarian cancer cells. |
Recipient cells undergo pro-tumorigenic pathway activation upon the transmission of oncogenic proteins, RNAs, and miRNAs. |
[31] |
Blood or genetic materials ( DNA etc.) |
Genetic profiling of GSTP1 may help find women who are at a higher risk of OC, which might lead to more targeted screening programs and better ways to avoid the disease. |
It aids in the elimination of electrophilic toxins by conjugating glutathione to them, hence decreasing their tendency to react. |
[32] |
|
Blood/ Genes |
It promotes folate absorption, which is essential for DNA formation, repair, and modification. In rapid-proliferating OC cells, increased FOLR1 expression increases folate bioavailability. |
This molecule exhibits a high-affinity binding to folate, subsequently facilitating its cellular internalization through the process of endocytosis. |
[33] |
|
Aldehyde Dehydrogenase 1 (ALDH1) |
Blood/ Cytosol of cells |
In OC, heightened ALDH1 expression exhibits a significant correlation with more advanced stages of the disease, tumors of higher grade, and diminished OS and PFS durations. |
ALDH1, an enzymatic catalyst, converts highly reactive aldehydes into less hazardous carboxylic acids. This metabolic pathway reduces oxidative stress-induced cell destruction. |
[34] |
PI3K/AKT/mTOR route
The PI3K/AKT/mTOR signalling axis represents the pivotal intracellular routes orchestrating cellular functions, encompassing growth, proliferation, differentiation, and survival [38, 39]. A multifaceted array of molecular mediators modulates this signalling cascade. These include various growth-promoting factors, notably IGF, EGF, and TGF. Additionally, the pathway is influenced by specific receptor tyrosine kinases, including IGF-1R, PDGFR, FGFR, HER2, and EGFR [40]. Furthermore, several associated receptor layers regulate this pathway [41, 42]. Evidence suggests a robust correlation between the anomalous expression and activation of AKT (pAKT) and diminished progression-free and overall survival outcomes in patients with epithelial OC [43, 44]. In high-grade serous OC, gene disruption frequently impairs the tumor-suppressing functions of RB1, PTEN, NF1, and RAD51B, as revealed by whole-genome sequencing. This genetic inactivation results in resistance to chemotherapy [45, 46]. The PI3K/PTEN/AKT signaling cascade is pivotal in directly modulating OC stemness, a critical regulatory element in aggressive malignancies. This modulation manifests in multiple ways, including augmentation of CSC populations, preservation of CSC phenotypic characteristics, and the emergence of multidrug resistance (MDR) mechanisms [47].
Experimental in vitro and in vivo studies have revealed the antineoplastic properties of SPR965, a compound that simultaneously targets the PI3K and mTORC1/2 signaling pathways. This dual inhibitor is effective against various solid malignancies, including serous ovarian neoplasms [48]. Nevertheless, additional clinical investigations are essential to validate its potential as a novel targeted therapeutic intervention before it can be recommended for clinical use. Further research has elucidated the role of ANGPTL3 in enhancing PTX sensitivity in OC by downregulating the PI3K-AKT-mTOR signaling pathway. The results of this investigation suggest that ANGPTL3 may emerge as a promising therapeutic target for OC, underscoring its potential significance in clinical strategies for managing this malignancy [49].
Apoptotic signaling pathway
Apoptosis, a meticulously regulated process of cellular self-destruction, is fundamental to maintaining equilibrium in multicellular organisms. This energy-dependent biochemical mechanism ensures a delicate balance between cell proliferation and elimination, thereby preserving overall tissue homeostasis [50, 51]. Regulation of mitochondrial membrane permeabilization, a critical process in the intrinsic apoptotic pathway, is orchestrated by two principal components: the B-cell lymphoma-2 (BCL-2) family and inhibitors of apoptotic proteins (IAPs). These molecular entities exert their influence by modulating caspase activation, thereby facilitating apoptotic switching [52]. In contrast, the extrinsic apoptotic pathway initiates a cell surface receptor signaling cascade via the tumor necrosis factor (TNF)-related apoptosis-inducing ligand (TRAIL) [53]. The activation of the caspase-3 pathway plays a pivotal role in the initiation of apoptosis. This phenomenon is demonstrated by the enhanced sensitivity to paclitaxel exhibited by human OC SKOV3.ip1 cells overexpressing HER-2/neu when exposed to adenoviral type 5 E1A [54]. Caspase-3 is a crucial enzyme that facilitates proteolytic degradation of cellular proteins and consequently advances the apoptotic cascade.
The polyphenolic compound resveratrol demonstrates the ability to enhance apoptotic processes via an AMPK-dependent pathway. Furthermore, this molecule facilitates caspase 3 activation, consequently leading to the downregulation of mTOR expression and activity [55]. In OC cells, mTOR is a downstream component of the AMPK signaling cascade. Enzastaurin functions as an ATP-competitive protein kinase C beta (PKC-beta) inhibitor and exhibits radiosensitizing properties, thus offering an alternative therapeutic approach. This compound suppresses tumor cell proliferation by amplifying the pro-apoptotic activities of caspase-3 and caspase-9 [56]. In human OVCAR-3 and OVCAR-4 cell lines, metformin's apoptotic effects are associated with several molecular changes. These include the reduction of Bcl-2 and Bcl-xL protein expression, the stimulation of caspase 3/7 activity, and the increase in Bax and Bad levels. Together, these alterations contribute to metformin's ability to trigger programmed cell death in these OC cells. Despite OVCAR-4 cells expressing Bcl-2, cisplatin increased metformin-induced apoptosis in OVCAR-3 cells without altering Bcl-2 levels [57].
The signaling pathway of JAK/STAT
Abnormal activation of the essential JAK/STAT signaling pathway is observed in OC. This ongoing stimulation significantly correlates with cancer development and unfavorable outcomes in individuals with these conditions [58]. In recent years, JAK inhibitors have become integral to cancer treatment. Clinical studies have demonstrated the effectiveness of various JAK inhibitors, and researchers are currently investigating additional inhibitors and related analogs for potential therapeutic applications. Preclinical investigations revealed that ruxolitinib, an FDA-approved JAK inhibitor for polycythemia vera treatment, effectively diminished OC cell viability [59]. In a murine model, the JAK inhibitor AZD1480, a small-molecule compound, demonstrated efficacy in suppressing OC growth [60]. This inhibition was mediated through cascading effects, including attenuation of STAT3 phosphorylation, disruption of DNA binding processes, and impediment of migration and adhesion in cultured OC cells [61].
Elevated aldehyde dehydrogenase (ALDH) activity, a hallmark of endometrial tumor stem cells, promotes the upregulation of interleukin-6 (IL-6) and its associated signal transducers, CD126 and GP130. In contrast, inhibition of the IL-6 receptor significantly suppresses the downstream IL-6/JAK1/STAT3 signaling pathway, ultimately reducing tumor cell proliferation [62]. In conclusion, many human cancers are associated with persistent activation of the JAK/STAT system, and the impact, if any, of JAK inhibitors on cancer progression is unclear. Therefore, the safety and efficacy of JAK inhibitors remain uncertain.
Autophagy has been identified as a potential therapeutic target in OC because it constitutively activates the PI3K/AKT/mTOR signaling pathway. This activation has been observed in approximately 70% of OC cases, highlighting the significance of autophagy in disease progression and treatment strategies [69]. Sirtuin 3 (Sirt3), a crucial member of the Sirtuin protein family, exhibits a fundamental role in preserving intracellular homeostasis and regulating autophagy in OC through intricate reciprocal interactions. Research has demonstrated that overexpression of Sirt3, induced by metformin, promotes mitochondrial impairment and programmed cell death in OC cells via AMPK activation [70].
To augment the effectiveness of cancer therapies, researchers have integrated a minimum of one chemotherapeutic compound into nanoparticles via encapsulation or incorporation techniques. Cisplatin, a prevalent first-line treatment for ovarian malignancies, encounters dosage constraints owing to its nephrotoxic effects [74]. In response to these challenges, scientific investigations have focused on optimizing cisplatin distribution and alleviating renal toxicity through advanced surface modification and nanoparticle engineering techniques [75]. The chemotherapeutic agent doxorubicin (Dox) is widely employed in the treatment of OC and breast cancer therapy. However, its clinical application is limited by severe cardiotoxicity. To address this issue, researchers have explored the encapsulation of doxorubicin in DDS. In a novel approach, Zeng et al. utilized the cucumber mosaic virus (CMV) as a biological scaffold to synthesize nanoparticles capable of controlled doxorubicin release, potentially mitigating its toxic effects [76].
The development of nano drug co-delivery systems (NDCDSs) represents a significant advancement in cancer treatment, particularly in chemotherapy. These innovative systems are designed to address the limitations of single-drug therapies by incorporating a minimum of two antitumor agents with distinct biophysical and pharmacological characteristics [77]. The primary objective of NDCDSs is to enhance the therapeutic efficacy while mitigating the toxic effects of conventional monotherapy approaches [78]. Advancements in siRNA-based drug codelivery systems have yielded promising results. A novel dendrimer, synthesized using polypropylenimine (PPI), has demonstrated efficacy in simultaneously transporting paclitaxel and a siRNA specifically targeting CD44 mRNA [79]. This hypothesis posited that inhibiting the cell surface CD44 marker through siRNA-mediated mechanisms would impede metastatic progression and enhance the efficacy of chemotherapeutic regimens. Nevertheless, concerns regarding the safety of prolonged administration arise from various factors, including biodegradation, bioavailability, instability, tissue distribution, and potential toxicity, which pose significant challenges.
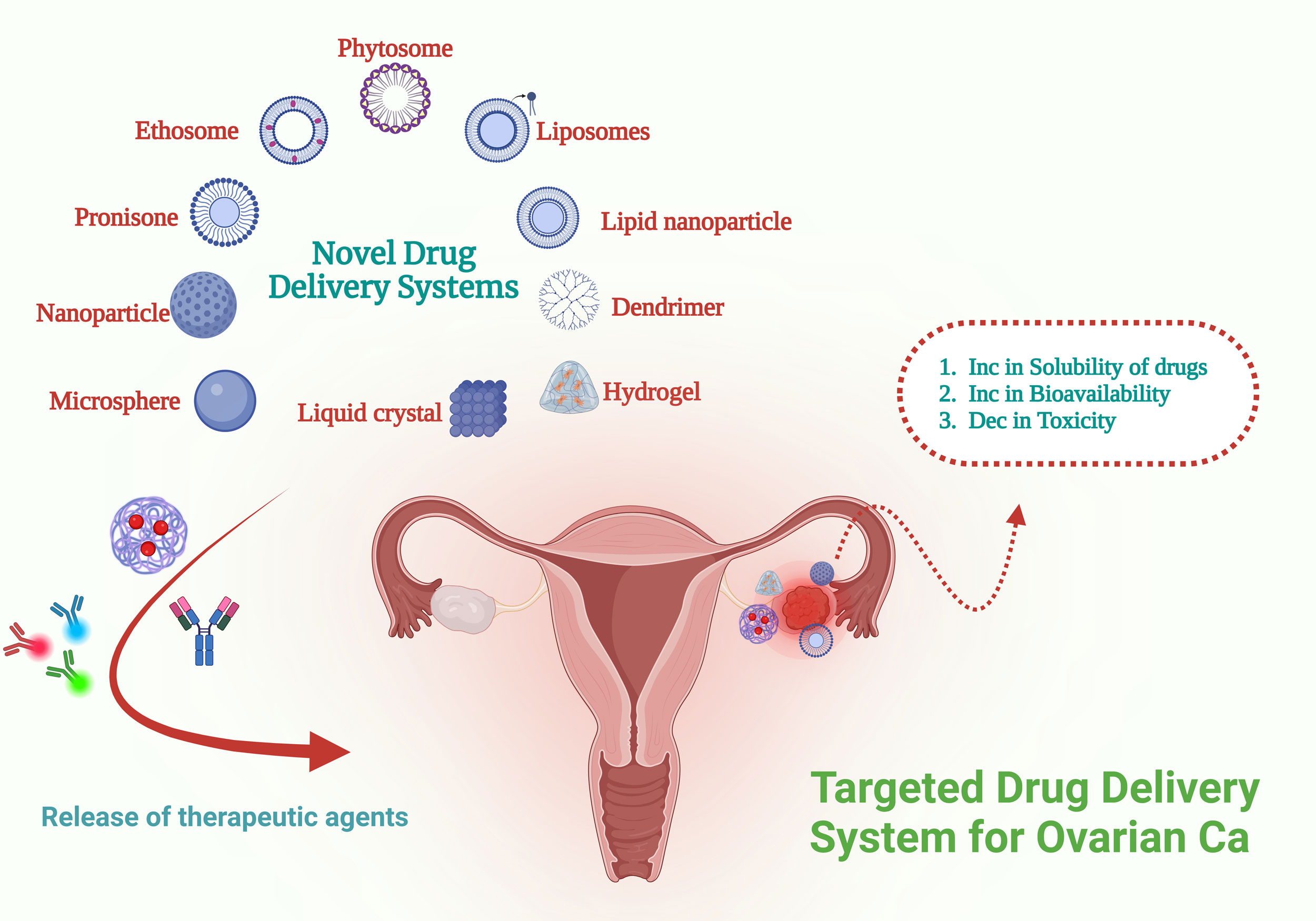
The signaling pathway of angiogenesis and VEFG
Angiogenesis, the biological process through which new blood vessels are generated, facilitates the transport of essential nutrients and oxygen to adjacent tissues [81]. This mechanism consequently supports the growth, invasive capabilities, and metastatic spread of tumor cells [82]. Recent scientific investigations have illuminated the pivotal role of receptor tyrosine kinases (RTKs) in aberrant blood vessel formation, particularly within the context of tumor-associated angiogenesis. Notably, vascular endothelial growth factor (VEGF), its cognate receptor (VEGFR), and the Flk-1/KDR RTK have emerged as critical regulators in this pathological process [83].
Amongst VEGF-targeting therapeutic approaches for OC, bevacizumab, an antibody directed against VEGF, is the most thoroughly researched intervention [84, 85]. Recent clinical trials evaluating bevacizumab in OC treatment yield encouraging results, particularly in deuce pivotal initial phase investigations: ICON7 [86] and GOG 218 [87]. The GOG trial employs a three-drug regimen, comprising carboplatin/paclitaxel and bevacizumab, for the treatment of OC patients who have undergone minimal cytoreductive procedures [88].
Targets particular to OC: CA125 and MUC16
Following its initial identification over twenty years ago, the CA125 antigen has been approved for clinical use in OC screening among high-risk women in the United States. Subsequent studies identified it as a promising prognostic biomarker for preinvasive OC [89]. Lloyd et al. successfully identified and characterized the gene encoding the CA125 antigen, which they subsequently designated as MUC16 [90]. Rab-B3.13, a monoclonal antibody from mice, alternatively called OvaRex, exhibits a robust binding affinity towards the CA125 antigen. Consequently, antibodies derived from murine sources, specifically targeting CA125, have recently been investigated as possible treatment methods. The section I/II clinical study demonstrated that patients with persistent OC established immunological responses to oregovomab and CA125 when administered as a third-line therapeutic intervention. The observed immune reactions included the production of antibodies and T cells. Notably, anti-idiotype antibodies were detected in 66% of the study participants [91]. The administration of specific anti-idiotypic antibodies as a vaccination strategy may potentially augment survival rates among patients with recurrent OC, demonstrating a favorable side effect profile. As a result, combining non-invasive immunotherapy techniques with standard chemotherapy protocols could potentially improve survival rates in OC patients [92].
Compounds targeting tubulin
For breast cancer and various other malignancies, including those of the ovaries, prostate, head, neck, and lungs, microtubule-targeting agents like taxane and vinca alkaloids have been utilized as frontline therapies for an extended period. These compounds belong to a class of anticancer drugs that exert their effects by interfering with microtubule function [93]. Clinical trials in phase III revealed that CT2103, a polyglutamated variant of paclitaxel, exhibited enhanced therapeutic efficacy and reduced adverse effects compared to conventional paclitaxel. This cytotoxic compound demonstrated improved clinical outcomes whilst minimizing undesirable patient reactions [94]. The revised paclitaxel formulation boasts quicker administration and reduced hypersensitivity reactions. It demonstrates taxane-equivalent effectiveness in recurring OC, yielding a 23% response rate among patients with minimal prior treatment [95]. Nevertheless, the medication's oral form exhibits poor absorption characteristics.
No applicable.
Ethics approval
No applicable.
Data availability
The data will be available upon request.
Funding
None.
Authors’ contribution
FTR contributed to the conception, design, writing of this review article and figures drawing. SN revised the review and submitted the final version of the manuscript.
Competing interests
None.
- Shih IM, Wang Y, Wang TL: The Origin of Ovarian Cancer Species and Precancerous Landscape. Am J Pathol 2021, 191(1): 26-39.
- Ahmed N, Kadife E, Raza A, Short M, Jubinsky PT, Kannourakis G: Ovarian Cancer, Cancer Stem Cells and Current Treatment Strategies: A Potential Role of Magmas in the Current Treatment Methods. Cells 2020, 9(3): 719.
- Lengyel E: Ovarian cancer development and metastasis. Am J Pathol 2010, 177(3): 1053-1064.
- Yang MQ, Elnitski L: A Systems Biology Comparison of Ovarian Cancers Implicates Putative Somatic Driver Mutations through Protein-Protein Interaction Models. PLoS One 2016, 11(10): e0163353.
- Bayraktar E, Chen S, Corvigno S, Liu J, Sood AK: Ovarian cancer metastasis: Looking beyond the surface. Cancer Cell 2024, 42(10): 1631-1636.
- Bast RC, Jr., Hennessy B, Mills GB: The biology of ovarian cancer: new opportunities for translation. Nat Rev Cancer 2009, 9(6): 415-428.
- Marcus CS, Maxwell GL, Darcy KM, Hamilton CA, McGuire WP: Current approaches and challenges in managing and monitoring treatment response in ovarian cancer. J Cancer 2014, 5(1): 25-30.
- van den Brand D, Mertens V, Massuger L, Brock R: siRNA in ovarian cancer - Delivery strategies and targets for therapy. J Control Release 2018, 283: 45-58.
- Fabbro M, Colombo PE, Leaha CM, Rouanet P, Carrère S, Quenet F, Gutowski M, Mourregot A, D'Hondt V, Coupier I, et al: Conditional Probability of Survival and Prognostic Factors in Long-Term Survivors of High-Grade Serous Ovarian Cancer. Cancers (Basel) 2020, 12(8): 2184.
- Siegel RL, Miller KD, Fuchs HE, Jemal A: Cancer statistics, 2022. CA Cancer J Clin 2022, 72(1): 7-33.
- Berek JS, Kehoe ST, Kumar L, Friedlander M: Cancer of the ovary, fallopian tube, and peritoneum. Int J Gynaecol Obstet 2018, 143 Suppl 2: 59-78.
- Feliu J, Heredia-Soto V, Gironés R, Jiménez-Munarriz B, Saldaña J, Guillén-Ponce C, Molina-Garrido MJ: Management of the toxicity of chemotherapy and targeted therapies in elderly cancer patients. Clin Transl Oncol 2020, 22(4): 457-467.
- Marchetti C, Pisano C, Facchini G, Bruni GS, Magazzino FP, Losito S, Pignata S: First-line treatment of advanced ovarian cancer: current research and perspectives. Expert Rev Anticancer Ther 2010, 10(1): 47-60.
- Webb PM, Jordan SJ: Epidemiology of epithelial ovarian cancer. Best Pract Res Clin Obstet Gynaecol 2017, 41: 3-14.
- Weiderpass E, Tyczynski JE: Epidemiology of Patients with Ovarian Cancer with and Without a BRCA1/2 Mutation. Mol Diagn Ther 2015, 19(6): 351-364.
- Stewart C, Ralyea C, Lockwood S: Ovarian Cancer: An Integrated Review. Semin Oncol Nurs 2019, 35(2): 151-156.
- La Vecchia C: Ovarian cancer: epidemiology and risk factors. Eur J Cancer Prev 2017, 26(1): 55-62.
- Khanlarkhani N, Azizi E, Amidi F, Khodarahmian M, Salehi E, Pazhohan A, Farhood B, Mortezae K, Goradel NH, Nashtaei MS: Metabolic risk factors of ovarian cancer: a review. JBRA Assist Reprod 2022, 26(2): 335-347.
- Webb PM, Jordan SJ: Global epidemiology of epithelial ovarian cancer. Nat Rev Clin Oncol 2024, 21(5): 389-400.
- Gaona-Luviano P, Medina-Gaona LA, Magaña-Pérez K: Epidemiology of ovarian cancer. Chin Clin Oncol 2020, 9(4): 47.
- Saleh M, Bhosale P, Menias CO, Ramalingam P, Jensen C, Iyer R, Ganeshan D: Ovarian teratomas: clinical features, imaging findings and management. Abdom Radiol (NY) 2021, 46(6): 2293-2307.
- Ali AT, Al-Ani O, Al-Ani F: Epidemiology and risk factors for ovarian cancer. Prz Menopauzalny 2023, 22(2): 93-104.
- Zamwar UM, Anjankar AP: Aetiology, Epidemiology, Histopathology, Classification, Detailed Evaluation, and Treatment of Ovarian Cancer. Cureus 2022, 14(10): e30561.
- Timmerman D, Testa AC, Bourne T, Ameye L, Jurkovic D, Van Holsbeke C, Paladini D, Van Calster B, Vergote I, Van Huffel S, et al: Simple ultrasound-based rules for the diagnosis of ovarian cancer. Ultrasound Obstet Gynecol 2008, 31(6): 681-690.
- Kaijser J, Bourne T, Valentin L, Sayasneh A, Van Holsbeke C, Vergote I, Testa AC, Franchi D, Van Calster B, Timmerman D: Improving strategies for diagnosing ovarian cancer: a summary of the International Ovarian Tumor Analysis (IOTA) studies. Ultrasound Obstet Gynecol 2013, 41(1): 9-20.
- Zhang M, Cheng S, Jin Y, Zhao Y, Wang Y: Roles of CA125 in diagnosis, prediction, and oncogenesis of ovarian cancer. Biochim Biophys Acta Rev Cancer 2021, 1875(2): 188503.
- Duan H, Liu X, Zhang Y, Liu Y, Ji Y, Zhang Y, Fan Z, Liu S, Yang L, Xu T, et al: Risk-stratified CA125 screening integrating CA125 trajectories, trajectory-specific progression and transvaginal ultrasound for ovarian cancer. J Ovarian Res 2024, 17(1): 210.
- Timmerman D, Van Calster B, Jurkovic D, Valentin L, Testa AC, Bernard JP, Van Holsbeke C, Van Huffel S, Vergote I, Bourne T: Inclusion of CA-125 does not improve mathematical models developed to distinguish between benign and malignant adnexal tumors. J Clin Oncol 2007, 25(27): 4194-4200.
- Jacobs IJ, Menon U, Ryan A, Gentry-Maharaj A, Burnell M, Kalsi JK, Amso NN, Apostolidou S, Benjamin E, Cruickshank D, et al: Ovarian cancer screening and mortality in the UK Collaborative Trial of Ovarian Cancer Screening (UKCTOCS): a randomised controlled trial. Lancet 2016, 387(10022): 945-956.
- Toubhans B, Gourlan AT, Telouk P, Lutchman-Singh K, Francis LW, Conlan RS, Margarit L, Gonzalez D, Charlet L: Cu isotope ratios are meaningful in ovarian cancer diagnosis. J Trace Elem Med Biol 2020, 62: 126611.
- McAlarnen LA, Gupta P, Singh R, Pradeep S, Chaluvally-Raghavan P: Extracellular vesicle contents as non-invasive biomarkers in ovarian malignancies. Mol Ther Oncolytics 2022, 26: 347-359.
- Simic P, Pljesa I, Nejkovic L, Jerotic D, Coric V, Stulic J, Kokosar N, Popov D, Savic-Radojevic A, Pazin V, et al: Glutathione Transferase P1: Potential Therapeutic Target in Ovarian Cancer. Medicina (Kaunas) 2022, 58(11): 1660.
- Gonzalez T, Muminovic M, Nano O, Vulfovich M: Folate Receptor Alpha-A Novel Approach to Cancer Therapy. Int J Mol Sci 2024, 25(2): 1046.
- Poturnajova M, Kozovska Z, Matuskova M: Aldehyde dehydrogenase 1A1 and 1A3 isoforms - mechanism of activation and regulation in cancer. Cell Signal 2021, 87: 110120.
- Terraneo N, Jacob F, Dubrovska A, Grünberg J: Novel Therapeutic Strategies for Ovarian Cancer Stem Cells. Front Oncol 2020, 10: 319.
- De Ruysscher D, Niedermann G, Burnet NG, Siva S, Lee AW, Hegi-Johnson F: Author correction: Radiotherapy toxicity. Nat Rev Dis Primers 2019, 5(1): 15.
- Yang L, Shi P, Zhao G, Xu J, Peng W, Zhang J, Zhang G, Wang X, Dong Z, Chen F, et al: Targeting cancer stem cell pathways for cancer therapy. Signal Transduct Target Ther 2020, 5(1): 8.
- Xia Q, Xu M, Zhang P, Liu L, Meng X, Dong L: Therapeutic Potential of Autophagy in Glioblastoma Treatment With Phosphoinositide 3-Kinase/Protein Kinase B/Mammalian Target of Rapamycin Signaling Pathway Inhibitors. Front Oncol 2020, 10: 572904.
- Driva TS, Schatz C, Haybaeck J: Endometriosis-Associated Ovarian Carcinomas: How PI3K/AKT/mTOR Pathway Affects Their Pathogenesis. Biomolecules 2023, 13(8): 1253.
- Hennessy BT, Smith DL, Ram PT, Lu Y, Mills GB: Exploiting the PI3K/AKT pathway for cancer drug discovery. Nat Rev Drug Discov 2005, 4(12): 988-1004.
- Mehta VB, Besner GE: HB-EGF promotes angiogenesis in endothelial cells via PI3-kinase and MAPK signaling pathways. Growth Factors 2007, 25(4): 253-263.
- Osaki M, Oshimura M, Ito H: PI3K-Akt pathway: its functions and alterations in human cancer. Apoptosis 2004, 9(6): 667-676.
- Cai J, Xu L, Tang H, Yang Q, Yi X, Fang Y, Zhu Y, Wang Z: The role of the PTEN/PI3K/Akt pathway on prognosis in epithelial ovarian cancer: a meta-analysis. Oncologist 2014, 19(5): 528-535.
- Huang TT, Lampert EJ, Coots C, Lee JM: Targeting the PI3K pathway and DNA damage response as a therapeutic strategy in ovarian cancer. Cancer Treat Rev 2020, 86: 102021.
- Patch AM, Christie EL, Etemadmoghadam D, Garsed DW, George J, Fereday S, Nones K, Cowin P, Alsop K, Bailey PJ, et al: Whole-genome characterization of chemoresistant ovarian cancer. Nature 2015, 521(7553): 489-494.
- Rinne N, Christie EL, Ardasheva A, Kwok CH, Demchenko N, Low C, Tralau-Stewart C, Fotopoulou C, Cunnea P: Targeting the PI3K/AKT/mTOR pathway in epithelial ovarian cancer, therapeutic treatment options for platinum-resistant ovarian cancer. Cancer Drug Resist 2021, 4(3): 573-595.
- Liu R, Chen Y, Liu G, Li C, Song Y, Cao Z, Li W, Hu J, Lu C, Liu Y: PI3K/AKT pathway as a key link modulates the multidrug resistance of cancers. Cell Death Dis 2020, 11(9): 797.
- Tran AQ, Sullivan SA, Chan LL, Yin Y, Sun W, Fang Z, Dugar S, Zhou C, Bae-Jump V: SPR965, a Dual PI3K/mTOR Inhibitor, as a Targeted Therapy in Ovarian Cancer. Front Oncol 2020, 10: 624498.
- Wu D, Liu J, Yang X, Wu Z, Wang T, Xiao M: ANGPTL3 diminishes the resistance of ovarian cancer to paclitaxel by blocking the PI3K-AKT-mTOR signaling pathway. Heliyon 2024, 10(11): e31520.
- Bertheloot D, Latz E, Franklin BS: Necroptosis, pyroptosis and apoptosis: an intricate game of cell death. Cell Mol Immunol 2021, 18(5): 1106-1121.
- Obeng E: Apoptosis (programmed cell death) and its signals - A review. Braz J Biol 2021, 81(4): 1133-1143.
- Kashyap D, Garg VK, Goel N: Intrinsic and extrinsic pathways of apoptosis: Role in cancer development and prognosis. Adv Protein Chem Struct Biol 2021, 125: 73-120.
- Huyghe J, Priem D, Bertrand MJM: Cell death checkpoints in the TNF pathway. Trends Immunol 2023, 44(8): 628-643.
- Ueno NT, Bartholomeusz C, Herrmann JL, Estrov Z, Shao R, Andreeff M, Price J, Paul RW, Anklesaria P, Yu D, et al: E1A-mediated paclitaxel sensitization in HER-2/neu-overexpressing ovarian cancer SKOV3.ip1 through apoptosis involving the caspase-3 pathway. Clin Cancer Res 2000, 6(1): 250-259.
- Liu Y, Tong L, Luo Y, Li X, Chen G, Wang Y: Resveratrol inhibits the proliferation and induces the apoptosis in ovarian cancer cells via inhibiting glycolysis and targeting AMPK/mTOR signaling pathway. J Cell Biochem 2018, 119(7): 6162-6172.
- Cadron I, Van Gorp T, Mihalyi A, Luyten C, Drijkoningen K, Amant F, Leunen K, Vergote I: The impact of enzastaurin (LY317615.HCl) on CA125 biosynthesis and shedding in ovarian cancer cells. Gynecol Oncol 2010, 118(1): 64-68.
- Yasmeen A, Beauchamp MC, Piura E, Segal E, Pollak M, Gotlieb WH: Induction of apoptosis by metformin in epithelial ovarian cancer: involvement of the Bcl-2 family proteins. Gynecol Oncol 2011, 121(3): 492-498.
- Wen W, Liang W, Wu J, Kowolik CM, Buettner R, Scuto A, Hsieh MY, Hong H, Brown CE, Forman SJ, et al: Targeting JAK1/STAT3 signaling suppresses tumor progression and metastasis in a peritoneal model of human ovarian cancer. Mol Cancer Ther 2014, 13(12): 3037-3048.
- Passamonti F, Palandri F, Saydam G, Callum J, Devos T, Guglielmelli P, Vannucchi AM, Zor E, Zuurman M, Gilotti G, et al: Ruxolitinib versus best available therapy in inadequately controlled polycythaemia vera without splenomegaly (RESPONSE-2): 5-year follow up of a randomised, phase 3b study. Lancet Haematol 2022, 9(7): e480-e492.
- Sun ZL, Tang YJ, Wu WG, Xing J, He YF, Xin DM, Yu YL, Yang Y, Han P: AZD1480 can inhibit the biological behavior of ovarian cancer SKOV3 cells in vitro. Asian Pac J Cancer Prev 2013, 14(8): 4823-4827.
- Hedvat M, Huszar D, Herrmann A, Gozgit JM, Schroeder A, Sheehy A, Buettner R, Proia D, Kowolik CM, Xin H, et al: The JAK2 inhibitor AZD1480 potently blocks Stat3 signaling and oncogenesis in solid tumors. Cancer Cell 2009, 16(6): 487-497.
- van der Zee M, Sacchetti A, Cansoy M, Joosten R, Teeuwssen M, Heijmans-Antonissen C, Ewing-Graham PC, Burger CW, Blok LJ, Fodde R: IL6/JAK1/STAT3 Signaling Blockade in Endometrial Cancer Affects the ALDHhi/CD126+ Stem-like Component and Reduces Tumor Burden. Cancer Res 2015, 75(17): 3608-3622.
- Rahman MA, Rahman MS, Rahman MDH, Rasheduzzaman M, Mamun-Or-Rashid A, Uddin MJ, Rahman MR, Hwang H, Pang MG, Rhim H: Modulatory Effects of Autophagy on APP Processing as a Potential Treatment Target for Alzheimer's Disease. Biomedicines 2020, 9(1): 5.
- Nokhostin F, Azadehrah M, Azadehrah M: The multifaced role and therapeutic regulation of autophagy in ovarian cancer. Clin Transl Oncol 2023, 25(5): 1207-1217.
- Feng C, Yuan X: Role of autophagy and its regulation by noncoding RNAs in ovarian cancer. Exp Biol Med (Maywood) 2023, 248(12): 1001-1012.
- Zhan L, Zhang Y, Wang W, Song E, Fan Y, Li J, Wei B: Autophagy as an emerging therapy target for ovarian carcinoma. Oncotarget 2016, 7(50): 83476-83487.
- Cai M, Hu Z, Liu J, Gao J, Liu C, Liu D, Tan M, Zhang D, Lin B: Beclin 1 expression in ovarian tissues and its effects on ovarian cancer prognosis. Int J Mol Sci 2014, 15(4): 5292-5303.
- Liang M, Zhao J: Protein expressions of AIB1, p53 and Bcl-2 in epithelial ovarian cancer and their correlations with the clinical pathological features and prognosis. Eur Rev Med Pharmacol Sci 2018, 22(16): 5134-5139.
- Orfanelli T, Jeong JM, Doulaveris G, Holcomb K, Witkin SS: Involvement of autophagy in cervical, endometrial and ovarian cancer. Int J Cancer 2014, 135(3): 519-528.
- Wu Y, Gao WN, Xue YN, Zhang LC, Zhang JJ, Lu SY, Yan XY, Yu HM, Su J, Sun LK: SIRT3 aggravates metformin-induced energy stress and apoptosis in ovarian cancer cells. Exp Cell Res 2018, 367(2): 137-149.
- Gidwani B, Vyas A: The potentials of nanotechnology-based drug delivery system for treatment of ovarian cancer. Artif Cells Nanomed Biotechnol 2015, 43(4): 291-297.
- Ye H, Karim AA, Loh XJ: Current treatment options and drug delivery systems as potential therapeutic agents for ovarian cancer: a review. Mater Sci Eng C Mater Biol Appl 2014, 45: 609-619.
- Manchester M, Singh P: Virus-based nanoparticles (VNPs): platform technologies for diagnostic imaging. Adv Drug Deliv Rev 2006, 58(14): 1505-1522.
- Lin F, Li X, Wang X, Sun H, Wang Z, Wang X: Stanniocalcin 1 promotes metastasis, lipid metabolism and cisplatin chemoresistance via the FOXC2/ITGB6 signaling axis in ovarian cancer. J Exp Clin Cancer Res 2022, 41(1): 129.
- Paraskar AS, Soni S, Chin KT, Chaudhuri P, Muto KW, Berkowitz J, Handlogten MW, Alves NJ, Bilgicer B, Dinulescu DM, et al: Harnessing structure-activity relationship to engineer a cisplatin nanoparticle for enhanced antitumor efficacy. Proc Natl Acad Sci U S A 2010, 107(28): 12435-12440.
- Zeng Q, Wen H, Wen Q, Chen X, Wang Y, Xuan W, Liang J, Wan S: Cucumber mosaic virus as drug delivery vehicle for doxorubicin. Biomaterials 2013, 34(19): 4632-4642.
- Qi SS, Sun JH, Yu HH, Yu SQ: Co-delivery nanoparticles of anti-cancer drugs for improving chemotherapy efficacy. Drug Deliv 2017, 24(1): 1909-1926.
- Li B, Shao H, Gao L, Li H, Sheng H, Zhu L: Nano-drug co-delivery system of natural active ingredients and chemotherapy drugs for cancer treatment: a review. Drug Deliv 2022, 29(1): 2130-2161.
- Shah V, Taratula O, Garbuzenko OB, Taratula OR, Rodriguez-Rodriguez L, Minko T: Targeted nanomedicine for suppression of CD44 and simultaneous cell death induction in ovarian cancer: an optimal delivery of siRNA and anticancer drug. Clin Cancer Res 2013, 19(22): 6193-6204.
- Banerjee S, Kaye SB: New strategies in the treatment of ovarian cancer: current clinical perspectives and future potential. Clin Cancer Res 2013, 19(5): 961-968.
- Lugano R, Ramachandran M, Dimberg A: Tumor angiogenesis: causes, consequences, challenges and opportunities. Cell Mol Life Sci 2020, 77(9): 1745-1770.
- Dudley AC, Griffioen AW: Pathological angiogenesis: mechanisms and therapeutic strategies. Angiogenesis 2023, 26(3): 313-347.
- Siveen KS, Prabhu K, Krishnankutty R, Kuttikrishnan S, Tsakou M, Alali FQ, Dermime S, Mohammad RM, Uddin S: Vascular Endothelial Growth Factor (VEGF) Signaling in Tumour Vascularization: Potential and Challenges. Curr Vasc Pharmacol 2017, 15(4): 339-351.
- Ferrara N, Hillan KJ, Gerber HP, Novotny W: Discovery and development of bevacizumab, an anti-VEGF antibody for treating cancer. Nat Rev Drug Discov 2004, 3(5): 391-400.
- Hall M, Gourley C, McNeish I, Ledermann J, Gore M, Jayson G, Perren T, Rustin G, Kaye S: Targeted anti-vascular therapies for ovarian cancer: current evidence. Br J Cancer 2013, 108(2): 250-258.
- Perren TJ, Swart AM, Pfisterer J, Ledermann JA, Pujade-Lauraine E, Kristensen G, Carey MS, Beale P, Cervantes A, Kurzeder C, et al: A phase 3 trial of bevacizumab in ovarian cancer. N Engl J Med 2011, 365(26): 2484-2496.
- Burger RA, Brady MF, Bookman MA, Fleming GF, Monk BJ, Huang H, Mannel RS, Homesley HD, Fowler J, Greer BE, et al: Incorporation of bevacizumab in the primary treatment of ovarian cancer. N Engl J Med 2011, 365(26): 2473-2483.
- Ray-Coquard I, Pautier P, Pignata S, Pérol D, González-Martín A, Berger R, Fujiwara K, Vergote I, Colombo N, Mäenpää J, et al: Olaparib plus Bevacizumab as First-Line Maintenance in Ovarian Cancer. N Engl J Med 2019, 381(25): 2416-2428.
- Gadducci A, Cosio S: Screening for Ovarian Cancer in the General Population: State of Art and Perspectives of Clinical Research. Anticancer Res 2022, 42(9): 4207-4216.
- Yin BW, Dnistrian A, Lloyd KO: Ovarian cancer antigen CA125 is encoded by the MUC16 mucin gene. Int J Cancer 2002, 98(5): 737-740.
- Frietze KM, Roden RB, Lee JH, Shi Y, Peabody DS, Chackerian B: Identification of Anti-CA125 Antibody Responses in Ovarian Cancer Patients by a Novel Deep Sequence-Coupled Biopanning Platform. Cancer Immunol Res 2016, 4(2): 157-164.
- Palaia I, Tomao F, Sassu CM, Musacchio L, Benedetti Panici P: Immunotherapy For Ovarian Cancer: Recent Advances And Combination Therapeutic Approaches. Onco Targets Ther 2020, 13: 6109-6129.
- Škubník J, Pavlíčková V, Ruml T, Rimpelová S: Current Perspectives on Taxanes: Focus on Their Bioactivity, Delivery and Combination Therapy. Plants (Basel) 2021, 10(3): 569.
- Langer CJ, O'Byrne KJ, Socinski MA, Mikhailov SM, Leśniewski-Kmak K, Smakal M, Ciuleanu TE, Orlov SV, Dediu M, Heigener D, et al: Phase III trial comparing paclitaxel poliglumex (CT-2103, PPX) in combination with carboplatin versus standard paclitaxel and carboplatin in the treatment of PS 2 patients with chemotherapy-naïve advanced non-small cell lung cancer. J Thorac Oncol 2008, 3(6): 623-630.
- Guastalla JP, 3rd, Diéras V: The taxanes: toxicity and quality of life considerations in advanced ovarian cancer. Br J Cancer 2003, 89 Suppl 3(Suppl 3): S16-22.
Asia-Pacific Journal of Oncology
print ISSN: 2708-7980, online ISSN: 2708-7999
Copyright © Asia Pac J Oncol. This work is licensed under a Creative Commons Attribution-NonCommercial-No Derivatives 4.0 International (CC BY-NC-ND 4.0) License.