Review | Open Access
Mechanisms of gut microbiota dysbiosis and colorectal cancer risk: analysis and prospective interventions
Dominic Kwesi Quainoo1, Nadiya Habib2
1Department of Biotechnology, Faculty of Biosciences, University for Development Studies, Ghana.
2Department of Zoology, University of Gujrat, Gujrat, Punjab, Pakistan.
Correspondence: Dominic Kwesi Quainoo (Department of Biotechnology, Faculty of Biosciences, University for Development Studies, Ghana; Email: dquainoo@uds.edu.gh).
Asia-Pacific Journal of Oncology 2024, 5: 23-31. https://doi.org/10.32948/ajo.2024.08.31
Received: 17 Aug 2024 | Accepted: 30 Aug 2024 | Published online: 05 Sep 2024
Key words gut microbiota dysbiosis, colorectal cancer, short-chain fatty acids, secondary bile acids, intestinal permeability, gene-environment interaction, dietary regulation, probiotics
Colorectal cancer (CRC) is one of the most common malignant tumors worldwide, with the incidence and mortality rates ranking the highest among malignant tumors [15-17]. According to global cancer statistics, over 1.9 million new CRC cases and more than 930,000 deaths are reported annually [18, 19]. Despite the recent advancements in early detection and survival rates of CRC screening and treatment techniques, its incidence continues to rise, especially in developing countries. The development of CRC is a complex process influenced by multiple factors, including genetic predisposition, dietary habits, lifestyle, and inflammatory responses [20, 21]. Recently, gut microbiota has emerged as a new research focus, garnering significant scholarly interest. Extensive research indicated a significant association between gut microbiota dysbiosis and the development of CRC. Specifically, dysbiosis may affect the course of CRC through mechanisms [22-25], such as the overgrowth of harmful bacteria and the accumulation of their metabolic byproducts, which may lead to the deterioration of the gut environment, inducing chronic inflammation and DNA damage in cells [26, 27]. Additionally, gut microbiota may influence CRC development by modulating the host immune system, altering intestinal permeability, and disrupting cellular signaling pathways. Although these findings offer valuable insights, the specific pathways remain unclear.
Against this backdrop, systematically analyzing the mechanisms linking gut microbiota dysbiosis with CRC risk and exploring potential intervention measures is of significant theoretical and clinical importance [28, 29]. On one hand, such analysis could elucidate CRC mechanisms, providing new perspectives for early diagnosis and prevention. On the other hand, interventions targeting gut microbiota regulation may emerge as novel strategies for CRC treatment. Therefore, this review aims to systematically discuss the potential roles of gut microbiota dysbiosis in CRC and its intervention prospects from multiple aspects, including gut microbiota composition and function, the mechanisms linking dysbiosis and CRC, and potential interventions. We aim to systematically review and analyze existing research findings to establish a robust reference base to inform and guide future research and clinical practice, thereby fostering progress in this field (Figure 1).
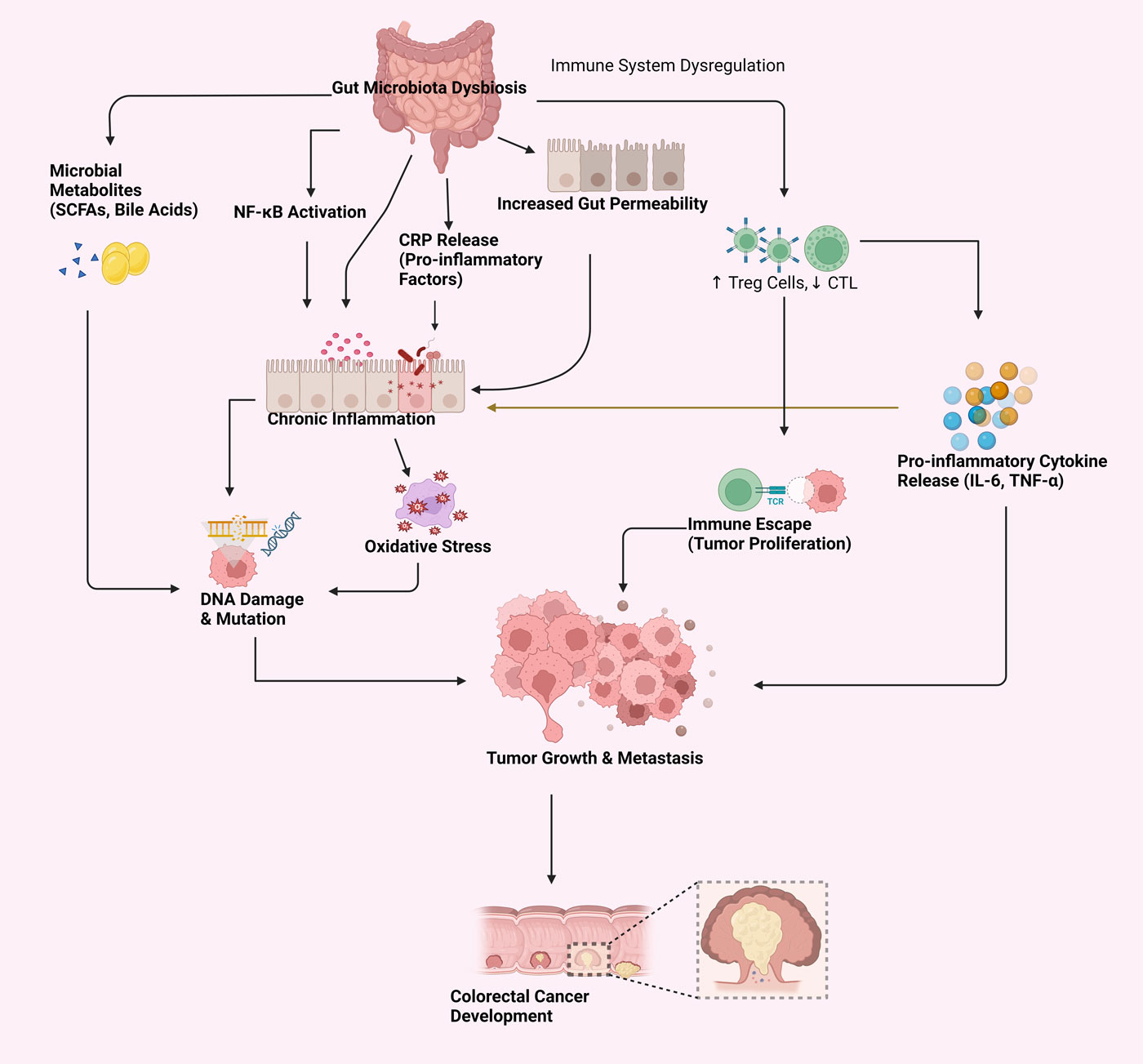
The normal gut microbiota is a complex microecosystem composed of bacteria, viruses, fungi, and other microorganisms [30-33]. Bacteria, the most abundant microorganism, are primarily divided into four major phyla based on their quantity and distribution in the gut: Firmicutes, Bacteroidetes, Actinobacteria, and Proteobacteria. Firmicutes and Bacteroidetes account for over 90% of the total gut microbiota, forming the core group that maintains gut microecological balance [34, 35].
Normal gut microbiota plays multiple essential roles in host health. It participates in the host's nutritional metabolism. For instance, gut microbiota can ferment undigested carbohydrates, producing short-chain fatty acids (SCFAs) such as acetate, propionate, and butyrate [36, 37]. These SCFAs provide energy for the host and regulate the gut's pH balance, inhibiting the growth of harmful microorganisms. Secondly, the gut microbiota is critical in maintaining the host's immune balance. Through interactions with the host's immune system, the normal gut microbiota can stimulate immune cells' development and functional maturation and regulate the host's immune responses, preventing excessive inflammatory reactions. Additionally, the microbiota enhances gut integrity by promoting the regeneration of intestinal epithelial cells, thereby sustaining normal gut permeability.
The figure illustrates how gut microbiota dysbiosis contributes to CRC risk through several interconnected mechanisms. Gut microbiota dysbiosis disrupts the balance of microbial metabolites, altering the short-chain fatty acids and generating toxic compounds that may induce carcinogenesis. Furthermore, this imbalance impacts gut permeability by compromising immune function and micro-movements within the gut barrier, thus fostering an environment prone to inflammation and cancer. Additionally, immune system modulation plays a critical role where immune homeostasis is disturbed, leading to immune activation and escape, particularly through mechanisms involving NF-κB activation. The dysregulated gut microbiome also facilitates immune evasion, permitting nascent cancer cells to avoid detection and enhance their proliferation. Collectively, these processes underscore the complex relationship between gut microbiota dysbiosis and CRC, highlighting multiple pathways through which an imbalanced microbiome can contribute to tumor development.
Definition and causes of gut microbiota imbalance
Gut microbiota imbalance, or dysbiosis, refers to abnormal changes in the composition and function of the gut microbiota. It is characterized by reduced beneficial bacteria, overgrowth of harmful bacteria, and reduced diversity and stability of gut microbiota. Several factors can lead to gut microbiota imbalance, including dietary habits, antibiotic use, infections, stress, lifestyle, and environmental changes [38, 39].
Diet is one of the primary factors influencing the composition and function of the gut microbiota. High-fat, high-sugar, and low-fiber diets can easily lead to gut microbiota imbalance. For instance, a high-fat diet increases bile acid secretion, fostering the growth of harmful sulfate-reducing bacteria in the gut. The metabolic products of these bacteria may damage the gut mucosa, leading to inflammation and disease. The widespread use of antibiotics is another significant factor causing gut microbiota imbalance. Huang K et al. found that antibiotics can kill pathogenic bacteria and severely damage beneficial bacteria in the gut, leading to opportunistic infections and overgrowth of harmful bacteria [40]. Additionally, factors such as acute or chronic intestinal infections, environmental pollution, irregular sleep patterns, and prolonged mental stress may directly or indirectly affect the composition and function of the gut microbiota, inducing dysbiosis.
Physiological and pathological impacts of gut microbiota imbalance
Gut microbiota dysbiosis profoundly affects the host's physiological and pathological states [41-45]. Firstly, it disrupts the host's metabolic balance, leading to the development of metabolic diseases. Studies, including the findings of Rutsch A et al., indicate a strong correlation between dysbiosis and conditions such as obesity, type 2 diabetes, and non-alcoholic fatty liver disease. Dysbiosis alters the host's energy metabolism, increases fat deposition, and affects insulin sensitivity, promoting these diseases [42]. Secondly, gut microbiota imbalance is closely related to various digestive system diseases. Inflammatory bowel diseases (IBD), such as Crohn's disease and ulcerative colitis, are closely associated with gut microbiota imbalance. Dysbiosis may contribute to the development and progression of chronic intestinal inflammation by disrupting the gut mucosal barrier and activating excessive immune responses.
Moreover, gut microbiota imbalance may increase the risk of CRC. The overgrowth of specific harmful bacteria, such as Escherichia coli and Clostridium, along with their toxic metabolic byproducts, can damage the DNA of intestinal epithelial cells, potentially inducing cancer. Gut microbiota imbalance also significantly affects the host's immune system. Moreover, dysbiosis significantly influences the immune system by abnormal activation or suppression, increasing susceptibility to infections and immune-mediated conditions such as allergies, rheumatoid arthritis, and systemic lupus erythematosus. Doroszkiewicz J et al. reported that dysbiosis affects the host's nervous system function through the gut-brain axis, leading to the development of mental and neurological disorders such as depression, autism, and Parkinson's disease [46].
The gut microbiota influences host health by metabolizing ingested food into various metabolites, including short-chain fatty acids (SCFAs), secondary bile acids, amines, indoles, and others. These metabolites can impact the occurrence and development of CRC through various mechanisms. For instance, harmful bacteria, such as sulfate-reducing bacteria and Enterococcus faecalis, can produce carcinogenic metabolites like hydrogen sulfide and nitrosamines, which can damage DNA and induce mutations [47, 48]. Moreover, microbial metabolites contribute to CRC risk by regulating the gut's redox balance and eliciting oxidative stress responses, thereby exacerbating the risk of carcinogenesis in intestinal epithelial cells.
The role of short-chain fatty acids (SCFAs)
SCFAs are metabolic byproducts produced by the gut microbiota through the fermentation of undigested carbohydrates, such as dietary fiber. The main SCFAs include acetate, propionate, and butyrate, which are important in maintaining gut health and regulating host metabolism [49, 50]. Butyrate, in particular, is extensively studied SCFAs in CRC prevention. It serves as a vital energy source for intestinal epithelial cells and exhibits anti-inflammatory, antioxidant, and anticancer properties. Butyrate can inhibit the occurrence and progression of CRC through various mechanisms. Firstly, it can inhibit the proliferation of CRC cells and promote their apoptosis. By inhibiting the activity of histone deacetylases (HDACs), butyrate increases the expression of specific tumor suppressor genes, thereby inhibiting cancer cell growth. Secondly, butyrate's anti-inflammatory actions are mediated through the activation of G-protein-coupled receptors (e.g., GPR43 and GPR109A), inhibiting the activation of the NF-κB signaling pathway, reducing the release of inflammatory mediators, and decreasing chronic inflammation [51, 52]. Additionally, butyrate enhances the gut mucosal barrier function, reducing gut permeability, preventing the entry of harmful substances into the body, and lowering the risk of inflammation and cancer.
However, gut microbiota imbalance reduces SCFA production, especially butyrate levels, which could be essential in developing CRC. Studies have shown that the relative abundance of Firmicutes and Bacteroidetes, the primary producers of SCFAs, is significantly reduced in CRC patients. Therefore, increasing dietary fiber intake to promote SCFA production may be a crucial strategy for preventing CRC.
Secondary bile acids and carcinogenic risk
Bile acids, synthesized by the liver and secreted into the intestine through bile, facilitate fat digestion. Primary bile acids are converted into secondary bile acids, such as deoxycholic acid (DCA) and lithocholic acid (LCA), by the gut microbiota [53-55]. Excessive accumulation of secondary bile acids in the gut may have carcinogenic effects. Research indicates a strong correlation between elevated levels of secondary bile acids and CRC development.
Secondary bile acids promote CRC through various pathways. Firstly, they induce oxidative stress responses in intestinal epithelial cells, leading to DNA damage and gene mutations, thereby increasing the risk of carcinogenesis. Secondly, secondary bile acids can activate the Wnt/β-catenin signaling pathway, a common carcinogenic pathway in CRC. Activation of this pathway can promote tumor cell proliferation and metastasis. Moreover, secondary bile acids can induce chronic inflammation by activating inflammatory signaling pathways such as the NF-κB pathway, further promoting cancer development. Therefore, reducing the accumulation of secondary bile acids or blocking their carcinogenic signaling pathways may be an important strategy for preventing and treating CRC.
Gut permeability and inflammatory response
Gut permeability refers to the intestinal barrier's ability to selectively regulate the passage of substances. Under normal conditions, the intestinal barrier can selectively absorb nutrients while preventing harmful substances, such as pathogens and toxins, from entering the body. However, Yu S et al. reported that gut microbiota imbalance may increase gut permeability, known as "leaky gut". This condition impairs the intestinal barrier, allowing harmful substances to infiltrate the body, provoking excessive immune responses and chronic inflammation [56]. Chronic inflammation is considered an important mechanism in the development of CRC.
Increased gut permeability can trigger a series of inflammatory responses, including the activation of macrophages and other immune cells, the release of pro-inflammatory factors, such as tumor necrosis factor-alpha (TNF-α), interleukin-6 (IL-6), and C-reactive protein (CRP) [57]. Bardelčíková AT et al. found that these inflammatory factors promote cancer cell proliferation, inhibit their apoptosis, and promote tumor angiogenesis and metastasis through various mechanisms. Additionally, chronic inflammation induces oxidative stress, further exacerbating DNA damage and gene mutations, increasing the risk of CRC [58]. Therefore, maintaining intestinal barrier integrity and reducing gut permeability is pivotal in preventing CRC. Interventions such as probiotics, prebiotics, and dietary fiber can regulate gut microbiota composition, enhance intestinal barrier function, reduce inflammatory responses, and lower the risk of CRC.
Alterations in the immune microenvironment
The gut microbiota influences the immune microenvironment within the tumor by interacting with the host's immune system, thereby affecting the occurrence and progression of CRC. The gut microbiota plays a role in immune surveillance by maintaining the balance of immune cells, including regulatory T cells (Tregs), Th17 cells, and cytotoxic T lymphocytes (CTLs) in the intestinal mucosa [59]. However, gut microbiota imbalance can disrupt this balance, leading to immune escape and promoting tumor development.
For example, the reduction in beneficial bacteria such as Bifidobacterium and Lactobacillus and the overgrowth of harmful bacteria can inhibit the activity of CTLs. Li Y e tal. reported that it reduces the immune system's ability to kill tumor cells and promote tumor immune escape [60]. On the other hand, gut microbiota imbalance can increase the proportion of immunosuppressive cells such as Tregs, inhibit the host's anti-tumor immune responses, and further promote tumor development. Additionally, gut microbiota imbalance can regulate immune responses by modulating the levels of immune-related signaling molecules, such as cytokines and chemokines, within the tumor microenvironment. Some harmful bacteria can secrete specific cytokines and chemokines, promoting the recruitment and activation of immune cells that suppress anti-tumor immunity and enhance tumor cell proliferation, invasion, and metastasis [61, 62].
Therefore, regulating gut microbiota composition and function may provide new therapeutic strategies for CRC. Interventions targeting gut microbiota, such as probiotics, prebiotics, and fecal microbiota transplantation, can potentially restore immune microenvironment balance, enhance anti-tumor immune responses, and improve CRC treatment outcomes.
The impact of antibiotics and the gut microbiota on CRC
The widespread use of antibiotics significantly impacts the composition and function of the gut microbiota, potentially influencing the risk of CRC. Antibiotics disrupt the balance by destroying the beneficial and harmful bacteria. Studies have shown that long-term or high-dose antibiotic use is associated with an increased risk of CRC [63-65].
Firstly, antibiotics reduce the gut microbiota diversity, leading to the overgrowth of specific harmful bacteria. These bacteria contribute to carcinogenesis by producing toxins, metabolites, and other toxic factors. Secondly, antibiotics can inhibit the production of beneficial metabolites, such as SCFAs, and weaken the protective effects of the gut microbiota on the intestinal barrier and immune system, thereby increasing the risk of cancer. Moreover, antibiotics induce gut microbiota imbalance, promoting chronic inflammation and immune dysregulation, further contributing to CRC development. Therefore, careful and appropriate use of antibiotics is essential for preventing gut microbiota imbalance and reducing CRC risk. Additionally, probiotics, prebiotics, and dietary adjustments may help restore gut microbiota balance and reduce the adverse effects of antibiotics on CRC risk.
Dietary regulation and improvement of gut microbiota
Diet is a key factor influencing the composition and function of gut microbiota. Proper dietary management can effectively improve the balance of gut microbiota and reduce the risk of CRC. Healthy nutritional components such as dietary fiber, plant polyphenols, and ω-3 fatty acids increase the abundance of beneficial bacteria, reduce the number of pathogenic bacteria, and restore the balance of the gut micro-ecosystem. For instance, a high-fibre diet promotes the production of short-chain fatty acids (SCFAs) like butyrate, enhances intestinal mucosal barrier function, reduces intestinal inflammation, and lowers the risk of CRC development [66]. Conversely, Mohseni AH et al. reported that a diet high in fat and sugar disrupts gut microbiota, increases the production of harmful metabolites such as secondary bile acids, and raises the likelihood of developing CRC [67]. Therefore, optimizing gut microbiota composition through dietary regulation is crucial for preventing CRC.
Application of prebiotics, probiotics, and synbiotics
The application of prebiotics, probiotics, and synbiotics significantly contributes to restoring the balance of gut microbiota and improves host health. Prebiotics, such as fructooligosaccharides and inulin, selectively foster beneficial bacteria like Bifidobacterium and Lactobacillus, enhancing gut microbiota composition. Probiotics, identified by Ashaolu TJ et al. as beneficial microorganisms like Bifidobacterium and Lactobacillus, improve gut microbiota balance and boost immune function [68]. Synbiotics, a combination of prebiotics and probiotics, further enhance the stability and diversity of gut microbiota through dual action. Research indicates that prebiotics and probiotics can significantly increase beneficial bacteria, reduce the growth of harmful bacteria, improve the gut microenvironment, and reduce the risk of CRC. Aindelis G. et al. found that probiotics offer anticancer benefits through pathogen exclusion, carcinogen production inhibition, intestinal barrier enhancement, and immune response regulation [69]. The application of synbiotics further enhances these benefits, offering new options for preventing and treating CRC.
Role of a high-fiber diet
A high-fiber diet is important in regulating gut microbiota and preventing CRC. Langfeld LQ et al. reported that dietary fiber, as a primary source of prebiotics, promotes the growth of beneficial bacterial communities like Bacteroidetes and Firmicutes in the gut and generates SCFAs (such as butyrate) through fermentation. The SCFAs are crucial in maintaining gut health, regulating inflammation, and immune responses [70]. A high-fiber diet increases stool bulk, shortens intestinal transit time, and reduces the contact time of harmful substances with the intestinal lining, lowering the risk of CRC. Additionally, dietary fiber can lower intestinal pH, inhibit the growth of pathogenic bacteria, and reduce the formation of carcinogenic substances, further decreasing cancer incidence. Nucci D et al. have shown that populations with high dietary fiber intake have a significantly lower incidence of CRC than those with low fiber intake [71]. Therefore, increasing dietary fiber prevents CRC effectively [71, 72].
Recovery of gut microbiota after antibiotic use
Antibiotics are crucial in treating infectious diseases, yet their impact on gut microbiota cannot be overlooked. The widespread use of antibiotics can alter gut microbiota imbalance, increase harmful bacteria proliferation, decrease beneficial bacteria, and subsequently increase the risk of CRC. Broad-spectrum antibiotics disrupt the microecological balance, increasing antibiotic-resistant strains and opportunistic pathogen infections. Therefore, restoring the balance of gut microbiota after antibiotic treatment is vital for preventing and treating CRC. Probiotics, prebiotics, and their combined use (synbiotics) effectively restore gut microbiota balance after antibiotic use. These substances effectively address the gut microbiota imbalance caused by antibiotics by promoting beneficial bacteria, suppressing harmful ones, and enhancing the intestinal barrier [73]. The rational use of antibiotics, including selecting appropriate antibiotics based on infection type and limiting unnecessary usage, is key to reducing gut microbiota imbalance and lowering the risk of CRC.
Application of fecal microbiota transplantation (FMT) in CRC prevention
Fecal microbiota transplantation (FMT) involves transplanting the gut microbiota from a healthy donor into a patient's intestine to restore balance. FMT has proven effective in treating refractory Clostridium difficile infections and is now being explored for CRC prevention. Restoring gut microbiota reduces the risk of CRC through various mechanisms [74]. Previous FMT has effectively restored the abundance of beneficial bacteria in the gut, reduced the number of pathogenic bacteria, and improved the gut microenvironment [75]. Additionally, FMT reduces the risk of inflammation and carcinogenesis by increasing SCFA production, enhancing intestinal mucosal barrier function, and regulating immune responses [76]. However, FMT efficacy in CRC prevention is still in the research stage, and further clinical studies are needed to verify its safety and efficacy.
Precision interventions targeting specific bacterial populations
As research on gut microbiota progresses, precision interventions targeting specific bacterial populations have become a new direction for CRC prevention. Sędzikowska A et al. found that gut microbiota composition varies significantly among individuals, and the overgrowth of specific carcinogenic bacteria may be an important cause of CRC [77]. Therefore, precision interventions aimed at specific bacterial populations offer the potential for more effective prevention and treatment. Based on metagenomics and microbiome analysis techniques, researchers can identify carcinogenic bacterial populations closely associated with CRC, such as specific strains of Escherichia coli and Clostridia. Precision intervention strategies may include selective use of antibiotics, targeted supplementation of probiotics, and regulation of specific metabolites. Additionally, personalized treatment plans based on the individual gut microbiota characteristics of patients may improve the prevention and treatment of CRC.
Prospects of novel therapeutic strategies
New therapeutic strategies deepen understanding of the relationship between gut microbiota and CRC. The combination of metabolic regulation and immunotherapy is at the forefront of CRC treatment. Modulating gut microbiota metabolites, such as increasing SCFA production and reducing secondary bile acid accumulation, alters the gut microenvironment and potentially decreases the incidence and progression of CRC [78]. Furthermore, the interaction between gut microbiota and the host immune system provides new insights for immunotherapy. The role of gut microbiota in immunotherapy has been preliminarily confirmed. Certain gut microbiota can enhance the host immune system's anti-tumor response, improving the efficacy of immunotherapeutic drugs like immune checkpoint inhibitors. Therefore, future research may enhance immunotherapy's effectiveness by modulating gut microbiota and developing more precise and personalized treatment plans. Overall, targeting gut microbiota presents substantial opportunities for preventing and treating CRC. Through strategies such as dietary regulation, application of prebiotics, probiotics, FMT, precision interventions, and exploration of novel therapeutic strategies, more comprehensive and effective solutions approaches are anticipated. Future research will further reveal the mechanisms and effects of these interventions, bringing new hope for the prevention and treatment of CRC.
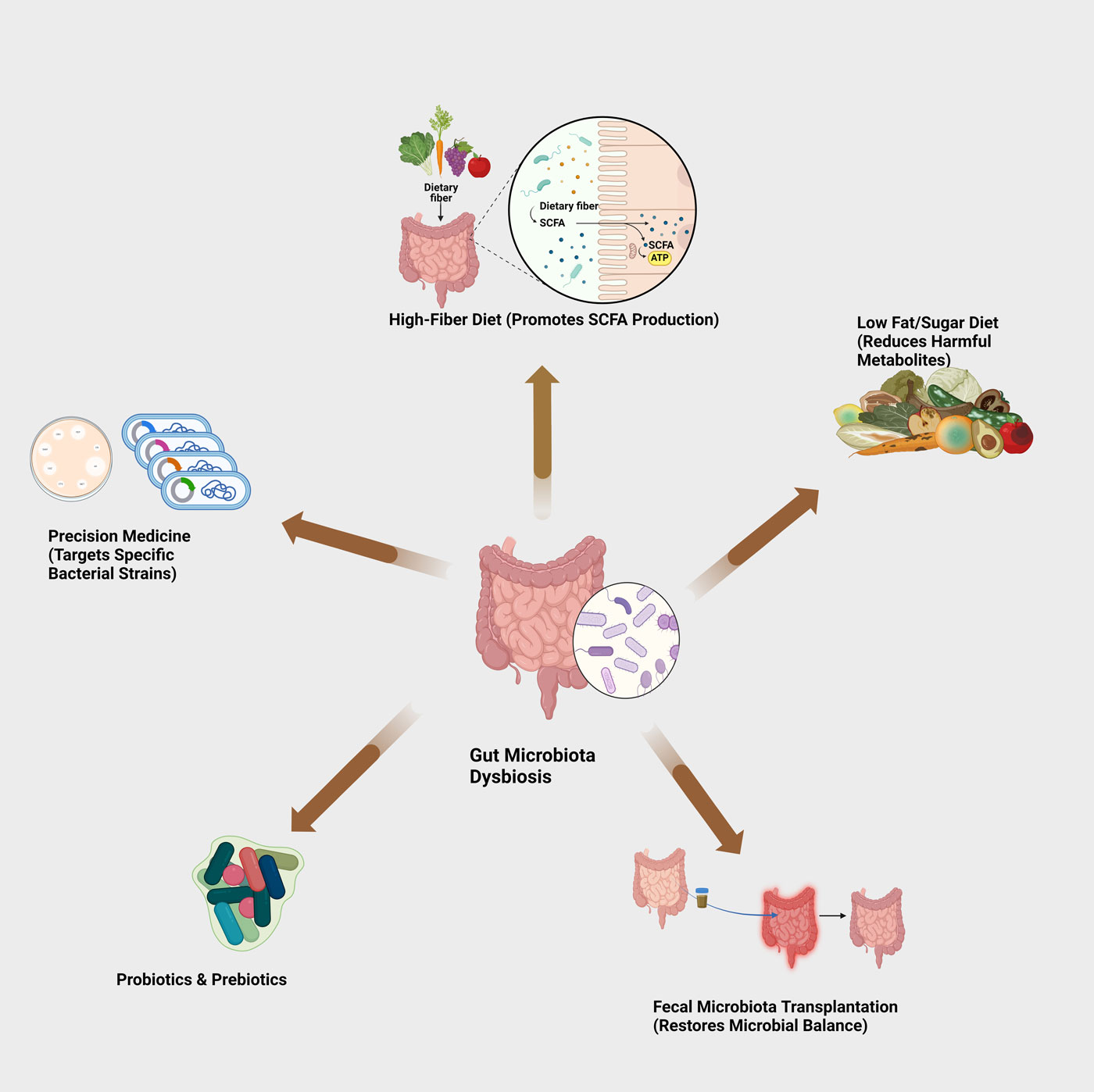
Despite recent advances in the study of gut microbiota and CRC, this field still faces numerous challenges. First, the diversity and complexity of the gut microbiota complicate understanding its role in CRC development. The gut microbiota is a complex ecosystem composed of hundreds of different microorganisms. Its function is influenced by the individual's genetic background and various external factors such as diet, environment, and medication use. Therefore, accurately deciphering the causal relationship between gut microbiota and CRC remains a key challenge for future research. Secondly, most current studies are cross-sectional or based on animal models, lacking long-term follow-up studies to verify the direct causal relationship. Additionally, inconsistencies across studies may stem from variations in study population, experimental design, and sample processing methods. Therefore, Standardizing research methodologies and conducting large-scale prospective studies are crucial to generating consistent, reliable results. Finally, the carcinogenic mechanisms of the gut microbiota in CRC are complex, involving microbial metabolism, inflammatory response, and immune regulation. Thus, comprehensively revealing the specific mechanisms by which gut microbiota contributes to the development of CRC through multi-level and multi-dimensional research approaches is also an important direction for future research.
The development prospects of personalized treatment and precision medicine
As research on the relationship between gut microbiota and CRC deepens, personalized treatment and precision medicine emerged as promising areas. The individualized nature of gut microbiota composition underlines the need to develop tailored prevention and treatment strategies. Future research is increasingly focused on personalizing approaches based on unique microbiota profiles. For example, by analyzing an individual's gut microbiota composition, identifying high-risk microbial groups, and then taking targeted intervention measures such as dietary adjustments, probiotics, or fecal microbiota transplantation, it is expected to significantly reduce the risk of CRC. Moreover, the development of precision medicine also relies on in-depth studies of the interaction between gut microbiota and host gene-environment interactions. Leveraging high-throughput techniques such as metagenomics, metabolomics, and immunomics allows researchers to delineate the mechanisms by which gut microbiota plays a role in personalized cancer prevention and treatment. This helps develop new biomarkers for early diagnosis and risk prediction and may promote the development of novel therapeutic strategies, such as precision immunotherapy and metabolic product regulation based on gut microbiota characteristics.
The importance of interdisciplinary research
Research on gut microbiota and CRC involves multiple disciplines, including microbiology, oncology, immunology, and metabolism, underscoring interdisciplinary research crucial for an in-depth understanding of its complex mechanisms. By integrating diverse knowledge and technical approaches, researchers can explore the role of gut microbiota in CRC from various angles. For instance, microbiological research helps identify carcinogenic microbial groups closely related to CRC. In contrast, oncological research can explore how these microbial groups influence tumor development through metabolic products, inflammatory responses, and other pathways. Immunology and metabolism studies also play important roles in the mechanistic links between gut microbiota and CRC. Researchers can develop more precise and effective intervention strategies by investigating how gut microbiota regulates the host immune response, influences the tumor microenvironment, and affects the growth and differentiation of tumor cells through metabolic products. Therefore, future research should emphasize collaborative efforts across disciplines. By promoting interdisciplinary research, we can advance the comprehensive understanding of the mechanisms linking gut microbiota and CRC and its clinical applications.
We would like to thank the participants of this study for sharing their experiences.
Availability of data and materials
Data and materials are available on request from the authors.
Ethical policy
Not applicable.
Author contributions
DKQ conceptualized, designed, conducted research, and wrote the manuscript; NH contributed to the revision and figure production.
Competing interests
I declare that there is no conflict of interest regarding the publication of this document. I confirm that neither I nor my collaborators have any financial or personal relationships that could inappropriately influence or bias the content of this work.
Funding
None.
- Gomaa EZ: Human gut microbiota/microbiome in health and diseases: a review. Antonie Van Leeuwenhoek 2020, 113(12): 2019-2040.
- Fan Y, Pedersen O: Gut microbiota in human metabolic health and disease. Nat Rev Microbiol 2021, 19(1): 55-71.
- Bhattarai S, Janaswamy S: The nexus of gut microbiota, diet, and health. Funct Food Sci 2022, 2(2): 47-63.
- Croci S, D'Apolito LI, Gasperi V, Catani MV, Savini I: Dietary strategies for the management of metabolic syndrome: role of gut microbiota metabolites. Nutrients 2021, 13(5): 1389.
- Yoo JY, Groer M, Dutra SVO, Sarkar A, McSkimming DI: Gut microbiota and immune system interactions. Microorganisms 2020, 8(10): 1587.
- Bajinka O, Darboe A, Tan Y, Abdelhalim KA, Cham LB: Gut microbiota and the human gut physiological changes. Ann Microbiol 2020, 70: 1-9.
- Vernocchi P, Del Chierico F, Putignani L: Gut microbiota metabolism and interaction with food components. Int J Mol Sci 2020, 21(10): 3688.
- Hrncir T: Gut microbiota dysbiosis: triggers, consequences, diagnostic and therapeutic options. Microorganisms 2022, 10(3): 578.
- Redondo-Useros N, Nova E, González-Zancada N, Díaz LE, Gómez-Martínez S, Marcos A: Microbiota and lifestyle: a special focus on diet. Nutrients 2020, 12(6): 1776.
- Martinez JE, Kahana DD, Ghuman S, Wilson HP, Wilson J, Kim SCJ, Lagishetty V, Jacobs JP, Sinha-Hikim AP, Friedman TC: Unhealthy lifestyle and gut dysbiosis: a better understanding of the effects of poor diet and nicotine on the intestinal microbiome. Front Endocrinol 2021, 12: 667066.
- Santana PT, Rosas SLB, Ribeiro BE, Marinho Y, de Souza HSP: Dysbiosis in inflammatory bowel disease: pathogenic role and potential therapeutic targets. Int J Mol Sci 2022, 23(7): 3464.
- Infante‐Villamil S, Huerlimann R, Jerry DR: Microbiome diversity and dysbiosis in aquaculture. Rev Aquacult 2021, 13(2): 1077-1096.
- Talapko J, Včev A, Meštrović T, Pustijanac E, Jukić M, Škrlec I: Homeostasis and dysbiosis of the intestinal microbiota: comparing hallmarks of a healthy state with changes in inflammatory bowel disease. Microorganisms 2022, 10(12): 2405.
- Arab JP, Arrese M, Shah VH: Gut microbiota in non‐alcoholic fatty liver disease and alcohol‐related liver disease: current concepts and perspectives. Hepatol Res 2020, 50(4): 407-418.
- Xi Y, Xu P: Global colorectal cancer burden in 2020 and projections to 2040. Transl Oncol 2021, 14(10): 101174.
- Wong MC, Huang J, Lok V, Wang J, Fung F, Ding H, Zheng ZJ: Differences in incidence and mortality trends of colorectal cancer worldwide based on sex, age, and anatomic location. Clin Gastroenterol Hepatol 2021, 19(5): 955-966.
- Zhou J, Zheng R, Zhang S, Zeng H, Wang S, Chen R, Sun K, Li M, Gu J, Zhuang G, Wei W: Colorectal cancer burden and trends: Comparison between China and major burden countries in the world. Chin J Cancer Res 2021, 33(1): 1-10.
- Gmeiner WH: Recent Advances in Therapeutic Strategies to Improve Colorectal Cancer Treatment. Cancers 2024, 16(5): 1029.
- Morgan E, Arnold M, Gini A, Lorenzoni V, Cabasag CJ, Laversanne M, Vignat J, Ferlay J, Murphy N, Bray F: Global burden of colorectal cancer in 2020 and 2040: incidence and mortality estimates from GLOBOCAN. Gut 2023, 72: 338-344.
- Puzzono M, Mannucci A, Grannò S, Zuppardo RA, Galli A, Danese S, Cavestro GM: The role of diet and lifestyle in early-onset colorectal cancer: a systematic review. Cancers 2021, 13(23): 5933.
- Schmitt M, Greten FR: The inflammatory pathogenesis of colorectal cancer. Nat Rev Immunol 2021, 21(10): 653-667.
- Janney A, Powrie F, Mann EH: Host–microbiota maladaptation in colorectal cancer. Nature 2020, 585(7826): 509-517.
- Zhao Y, Jiang Q: Roles of the polyphenol–gut microbiota interaction in alleviating colitis and preventing colitis-associated colorectal cancer. Adv Nutr 2021, 12(2): 546-565.
- Cheng Y, Ling Z, Li L: The intestinal microbiota and colorectal cancer. Front Immunol 2020, 11: 615056.
- Niekamp P, Kim CH: Microbial metabolite dysbiosis and colorectal cancer. Gut liver 2023, 17(2): 190-203.
- Sarkar K, Sil PC: Effect of diet, pharmaceuticals, and environmental toxicants on gut microbiota imbalance and increased intestinal membrane permeability. In: Toxicological Risk Assessment and Multi-System Health Impacts from Exposure. Academic Press, 2021: 403-413.
- Glorieux G, Gryp T, Perna A: Gut-derived metabolites and their role in immune dysfunction in chronic kidney disease. Toxins 2020, 12(4): 245.
- Fong W, Li Q, Yu J: Gut microbiota modulation: a novel strategy for prevention and treatment of colorectal cancer. Oncogene 2020, 39(26): 4925-4943.
- Wang Z, Dan W, Zhang N, Fang J, Yang Y: Colorectal cancer and gut microbiota studies in China. Gut Microbes 2023, 15(1): 2236364.
- Pérez JC: Fungi of the human gut microbiota: Roles and significance. Int J Med Microbiol 2021, 311(3): 151490.
- Chen L, Wang J: Gut microbiota and inflammatory bowel disease. WIREs Mech Dis 2022, 14(2): e1540.
- Chen Y, Zhou J, Wang L: Role and mechanism of gut microbiota in human disease. Front Cell Infect Microbiol 2021, 11: 625913.
- Bilgin B, Hancı H: Gut Microbiota and Its Importance for Our Health. Pharmata 2023, 3(3): 71-73.
- Bozomitu L, Miron I, Adam Raileanu A, Lupu A, Paduraru G, Marcu FM, Buga AML, Rusu DC, Dragan F, Lupu VV: The Gut Microbiome and Its Implication in the Mucosal Digestive Disorders. Biomedicines 2022, 10(12): 3117.
- Álvarez-Mercado AI, Plaza-Diaz J: Dietary polysaccharides as modulators of the gut microbiota ecosystem: An update on their impact on health. Nutrients 2022, 14(19): 4116.
- Ashaolu TJ, Ashaolu JO, Adeyeye SAO: Fermentation of prebiotics by human colonic microbiota in vitro and short‐chain fatty acids production: a critical review. J Appl Microbiol 2021, 130(3): 677-687.
- Cong J, Zhou P, Zhang R: Intestinal microbiota-derived short chain fatty acids in host health and disease. Nutrients 2022, 14(9): 1977.
- Anderson KM, Ferranti EP, Alagha EC, Mykityshyn E, French CE, Reilly CM: The heart and gut relationship: a systematic review of the evaluation of the microbiome and trimethylamine-N-oxide (TMAO) in heart failure. Heart Fail Rev 2022, 27(6): 2223-2249.
- Nova E, Gómez-Martínez S, González-Soltero R: The influence of dietary factors on the gut microbiota. Microorganisms 2022, 10(7): 1368.
- de Nies L, Kobras CM, Stracy M: Antibiotic-induced collateral damage to the microbiota and associated infections. Nat Rev Microbiol 2023, 21(12): 789-804.
- Huang K, Wu L, Yang Y: Gut microbiota: An emerging biological diagnostic and treatment approach for gastrointestinal diseases. JGH Open 2021, 5(9): 973-975.
- Rutsch A, Kantsjö JB, Ronchi F: The gut-brain axis: how microbiota and host inflammasome influence brain physiology and pathology. Front Immunol 2020, 11: 604179.
- Andoh A: Physiological role of gut microbiota for maintaining human health. Dig Dis 2019, 36(3): 176-181.
- Jones RM, Neish AS: Gut microbiota in intestinal and liver disease. Annu Rev Pathol 2021, 16(1): 251-275.
- Deidda G, Biazzo M: Gut and brain: investigating physiological and pathological interactions between microbiota and brain to gain new therapeutic avenues for brain diseases. Front Neurosci 2021, 15: 753915.
- Doroszkiewicz J, Groblewska M, Mroczko B: The role of gut microbiota and gut–brain interplay in selected diseases of the central nervous system. Int J Mol Sci 2021, 22(18): 10028.
- Balmain A: The critical roles of somatic mutations and environmental tumor-promoting agents in cancer risk. Nat Genet 2020, 52(11): 1139-1143.
- Zhu Y, Costa M: Metals and molecular carcinogenesis. Carcinogenesis 2020, 41(9): 1161-1172.
- Ranjbar R, Vahdati SN, Tavakoli S, Khodaie R, Behboudi H: Immunomodulatory roles of microbiota-derived short-chain fatty acids in bacterial infections. Biomed Pharmacother 2021, 141: 111817.
- Kim CH: Control of lymphocyte functions by gut microbiota-derived short-chain fatty acids. Cell Mol Immunol 2021, 18(5): 1161-1171.
- Chen J, Vitetta L: The role of butyrate in attenuating pathobiont-induced hyperinflammation. Immune Netw 2020, 20(2): e15.
- Liu P, Wang Y, Yang G, Zhang Q, Meng L, Xin Y, Jiang X: The role of short-chain fatty acids in intestinal barrier function, inflammation, oxidative stress, and colonic carcinogenesis. Pharmacol Res 2021, 165: 105420.
- Kiriyama Y, Nochi H: The Role of Gut Microbiota-Derived Lithocholic Acid, Deoxycholic Acid and Their Derivatives on the Function and Differentiation of Immune Cells. Microorganisms 2023, 11(11): 2730.
- Yang R, Qian L: Research on gut microbiota-derived secondary bile acids in cancer progression. Integr Cancer Ther 2022, 21: 15347354221114100.
- Godlewska U, Bulanda E, Wypych TP: Bile acids in immunity: Bidirectional mediators between the host and the microbiota. Front Immunol 2022, 13: 949033.
- Yu S, Sun Y, Shao X, Zhou Y, Yu Y, Kuai X, Zhou C: Leaky Gut in IBD: Intestinal Barrier-Gut Microbiota Interaction. J Microbiol Biotechnol 2022, 32(7): 825-834.
- Olszewska E, Pietrewicz TM, Świderska M, Jamiołkowski J, Chabowski A: A case-control study on the changes in high-sensitivity C-reactive protein and tumor necrosis factor-alpha levels with surgical treatment of OSAS. Int J Mol Sci 2022, 23(22): 14116.
- Bardelčíková A, Šoltys J, Mojžiš J: Oxidative stress, inflammation and colorectal cancer: an overview. Antioxidants 2023, 12(4): 901.
- Cosola C, Rocchetti MT, Gesualdo L: Gut microbiota, the immune system, and cytotoxic T lymphocytes. In: Cytotoxic T-Cells: Methods and Protocols 2021, 2325: 229-241.
- Li Y, Ye Z, Zhu J, Fang S, Meng L, Zhou C: Effects of gut microbiota on host adaptive immunity under immune homeostasis and tumor pathology state. Front Immunol 2022, 13: 844335.
- Aghamajidi A, Maleki Vareki S: The effect of the gut microbiota on systemic and anti-tumor immunity and response to systemic therapy against cancer. Cancers 2022, 14(15): 3563.
- Huang YK, Busuttil RA, Boussioutas A: The role of innate immune cells in tumor invasion and metastasis. Cancers 2021, 13(23): 5885.
- Queen J, Zhang J, Sears CL: Oral antibiotic use and chronic disease: long-term health impact beyond antimicrobial resistance and Clostridioides difficile. Gut Microbes 2020, 11(4): 1092-1103.
- Nadeem MS, Kumar V, Al-Abbasi FA, Kamal MA, Anwar F: Risk of colorectal cancer in inflammatory bowel diseases. Semin Cancer Biol 2020, 64: 51-60.
- Raoul JL, Edeline J, Simmet V, Moreau-Bachelard C, Gilabert M, Frénel JS: Long-term use of proton pump inhibitors in cancer patients: an opinion paper. Cancers 2022, 14(5): 1156.
- Magliocca G, Mone P, Di Iorio BR, Heidland A, Marzocco S: Short-chain fatty acids in chronic kidney disease: Focus on inflammation and oxidative stress regulation. Int J Mol Sci 2022, 23(10): 5354.
- Mohseni AH, Taghinezhad-SS, Fu X: Gut microbiota-derived metabolites and colorectal cancer: New insights and updates. Microb Pathog 2020, 149: 104569.
- Ashaolu TJ: Immune boosting functional foods and their mechanisms: A critical evaluation of probiotics and prebiotics. Biomed Pharmacother 2020, 130: 110625.
- Aindelis G, Chlichlia K: Modulation of anti-tumour immune responses by probiotic bacteria. Vaccines 2020, 8(2): 329.
- Langfeld LQ, Du K, Bereswill S, Heimesaat MM: A review of the antimicrobial and immune-modulatory properties of the gut microbiota-derived short chain fatty acid propionate—What is new? Eur J Microbiol Immunol 2021, 11(2): 50-56.
- Nucci D, Fatigoni C, Salvatori T, Nardi M, Realdon S, Gianfredi V: Association between dietary fibre intake and colorectal adenoma: A systematic review and meta-analysis. Int J Environ Res Public Health 2021, 18(8): 4168.
- Pedrosa LF, Fabi JP: Dietary fiber as a wide pillar of colorectal cancer prevention and adjuvant therapy. Crit Rev Food Sci Nutr 2024, 64(18): 6177-6197.
- Wang X, Zhang P, Zhang X: Probiotics regulate gut microbiota: an effective method to improve immunity. Molecules 2021, 26(19): 6076.
- Wong CC, Yu J: Gut microbiota in colorectal cancer development and therapy. Nat Rev Clin Oncol 2023, 20(7): 429-452.
- Airola C, Severino A, Porcari S, Fusco W, Mullish BH, Gasbarrini A, Cammarota G, Ponziani FR, Ianiro G: Future Modulation of Gut Microbiota: From Eubiotics to FMT, Engineered Bacteria, and Phage Therapy. Antibiotics 2023, 12(5): 868.
- Li X, Zhang S, Guo G, Han J, Yu J: Gut microbiome in modulating immune checkpoint inhibitors. EBioMedicine 2022, 82: 104163.
- Sędzikowska A, Szablewski L: Human gut microbiota in health and selected cancers. Int J Mol Sci 2021, 22(24): 13440.
- Xu R, Wang Q, Li L: A genome-wide systems analysis reveals strong link between colorectal cancer and trimethylamine N-oxide (TMAO), a gut microbial metabolite of dietary meat and fat. BMC Genom 2015, 16 Suppl 7(Suppl 7): S4.
Asia-Pacific Journal of Oncology
print ISSN: 2708-7980, online ISSN: 2708-7999
Copyright © Asia Pac J Oncol. This work is licensed under a Creative Commons Attribution-NonCommercial-No Derivatives 4.0 International (CC BY-NC-ND 4.0) License.