Review | Open Access
Progress in research and treatment of immune checkpoints in breast cancer
Xinyi Zhang1, *, Jie Wang2, *, Kaiyuan Zhou3
1Quality Control Department, Shaanxi Provincial Hospital of Traditional Chinese Medicine, Xi’an, PR. China.
2Department of Otolaryngology-Head and Neck Surgery, The Affiliated Children Hospital of Xi’an Jiaotong University, Xi’an, PR. China.
3Health Service Department, Air Force Medical University, Xi’an, PR. China.
*: Xinyi Zhang and Jie Wang equally contribute to the research works.
Correspondence: Kaiyuan Zhou (Health Service Department, Air Force Medical University, 169 Changle West Street, Xi’an, 710032, PR. China; Email: csnrzky@126.com).
Asia-Pacific Journal of Oncology 2024, 5: 9-15. https://doi.org/10.32948/ajo.2024.04.06
Received: 05 Mar 2024 | Accepted: 02 Apr 2024 | Published online: 08 Apr 2024
Key words breast cancer, immune checkpoint, targeted therapy, review
Tumor cells employ diverse strategies to evade immune surveillance include immunoediting to alter immunogenicity [1] and exploitation of tumor microenvironment to evade recognition and elimination by the immune system [2]. A range of anti-tumor immune mechanisms have been discovered including tumor vaccines that enhance antigen recognition by the immune system, adoptive cell therapy which supplements cytotoxic immune cells, and immunotherapy targeting inhibitory checkpoints on T-cells to counter immunosuppression [3].
The use of immune checkpoint inhibitors (ICI) in breast cancer treatment has shown significant progress; however, the gene expression patterns of immune checkpoints vary, and the therapeutic evaluation values of different immune checkpoints in tumors also differ. In this review article, we provide an overview of general aspects related to immune checkpoints and explore recent advancements in strategies to suppress these checkpoints for treatment of breast cancer. Additionally, we highlighted recent research breakthrough concerning breast cancer-related immune checkpoints.
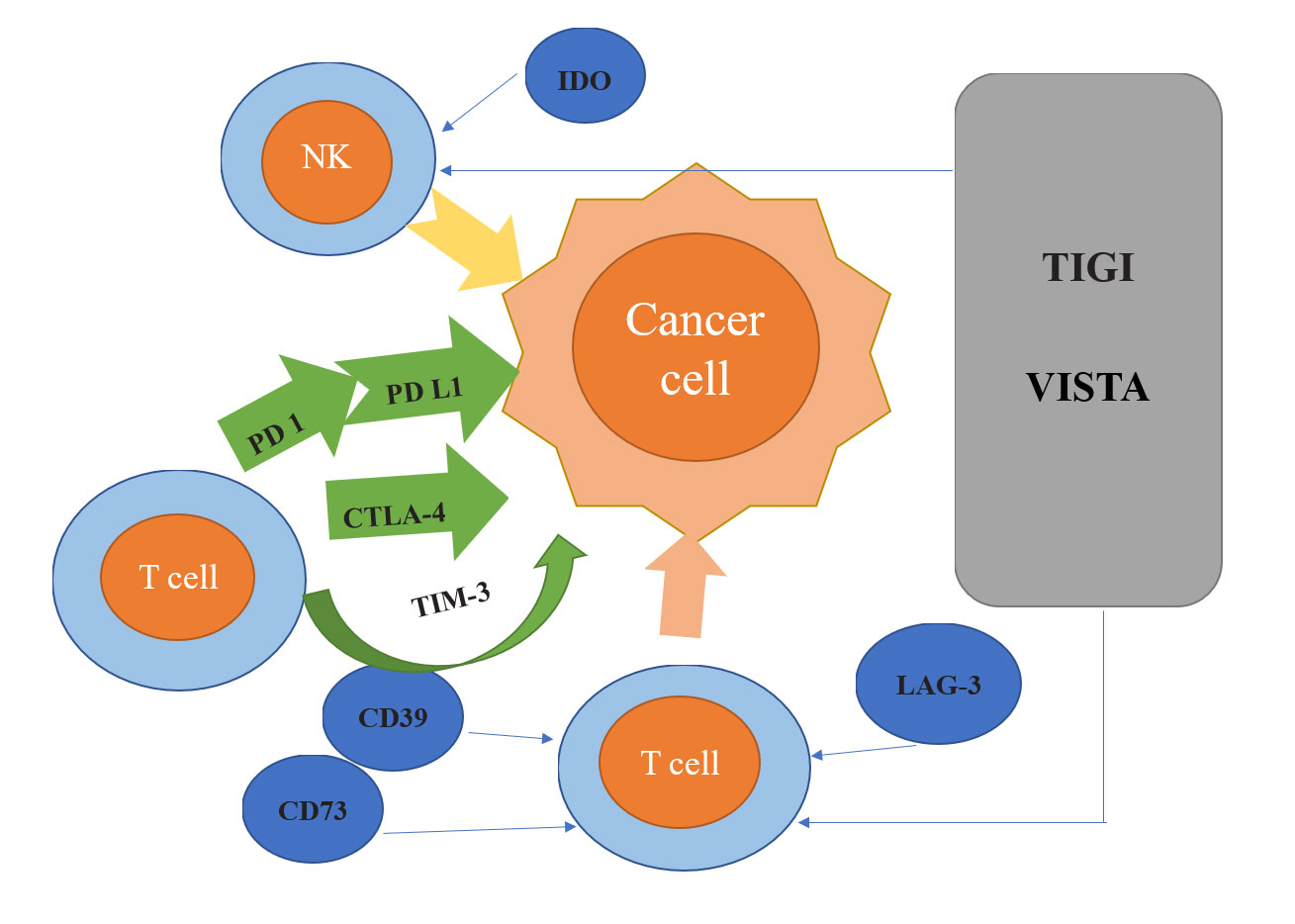
Programmed cell death protein 1(PD-1)
PD-1, a class of inhibitory regulatory receptors on the surface of T cells, belongs to the immunoglobulin superfamily type I transmembrane glycoprotein and usually expressed on active cells such as T cells, natural killer cells, B cells, and macrophages [10]. Structurally, PD-1 is a type I transmembrane protein featuring a cytoplasmic domain containing an immunoreceptor tyrosine-based inhibitory motif (ITIM) and an immunoreceptor tyrosine-based switch motif (ITSM) [11]. Its inhibitory effects extend to NK cell cytotoxicity and cytokine production [12].
In BC, PD-1 expression levels correlate with T stage, age, and the Ki-67 proliferation index. Elevated PD-1 expression is associated with shorter overall survival and progression-free survival in BC patients, with higher expression levels observed in most BC subtypes except for Luminal A BC. Notable inhibitors targeting PD-1 in BC treatment include Pembrolizumab, Nivolumab, Atezolizumab, and Camrelizumab, among others. Attillizumab, the first immune checkpoint inhibitor approved for triple-negative breast cancer ITNBC) treatment, can be combined with chemotherapy for locally advanced unresectable cases [13]. Voorwerk demonstrated a higher objective remission rate in patients treated with the combination of nebuliuzumab and chemotherapy, along with the up-regulation of immune-related genes involved in the PD-1/PD-L1 signaling pathway and T cell-mediated immune killing [14]. Wu evaluated the feasibility of combining chemotherapy with carrilizumab and Famitinib in patients with advanced TNBC [15].
Programmed cell death 1 ligand 1(PD-L1)
The primary ligand of the immunosuppressive receptor PD-1 plays a crucial role in modulating immune responses, exerting a negative regulatory function [16]. PD-L1 is predominantly expressed on the surface of various solid tumor cells, including lung cancer, malignant melanoma, ovarian cancer, prostate cancer, and BC [17]. Apart from tumor cells, PD-L1 can also be detected in other components of the tumor microenvironment such as tumor-infiltrating lymphocytes (TILs) and macrophages [18]. Currently, immunohistochemical methods are primarily used for PD-L1 detection in BC, although standardization regarding the choice of antibodies and positive criteria remains lacking.
In BC, PD-L1 expression levels typically correlate with the presence of TILs and clinicopathological features associated with adverse outcomes. It includes younger age, ductal histology, large tumor size, estrogen receptor (ER) negativity, progesterone receptor (PR) negativity, human epidermal growth factor receptor 2 (HER2) positivity, high proliferation rate, and aggressive molecular subtypes. A meta-analysis of 37 studies examining PD-L1 expression across different BC subtypes revealed elevated expression in TNBC and HER2 amplification subtypes [19]. Several neoadjuvant therapy trials have identified high PD-L1 expression as an independent predictor of favorable pathological response at both mRNA and protein levels [20-21]. However, a study involving 870 BC patients reported that high PD-L1 expression was associated with lower disease-free survival (DFS) and metastasis-free survival (MFS), indicating a poor prognosis for these patients [22]. Conversely, another study involving 636 stage I-III BC patients found that PD-L1 mRNA expression was linked to improved recurrence-free survival (RFS) [23]. A meta-analysis focusing on Luminal A, Luminal B, HER2-based amplification, and TNBC subtypes indicated that while PD-L1 expression correlated with shorter overall survival (OS) and DFS, this association was absent within the TNBC subtype [24]. Notably, in several studies specifically targeting TNBC or basal-like patterns, PD-L1 expression predicted higher OS rates [25-27].
Cytotoxic T lymphocyte-associated antigen-4(CTLA-4)
CTLA4, a transmembrane protein expressed on both CD4+ and CD8+ T cells, functions to inhibit T cell activation through two mechanisms. Firstly, by binding to the B7 ligand on the antigen-presenting cell (APC) surface, CTLA-4 competitively inhibits CD28 co-stimulatory signals, thereby exerting an extracellular space-occupying effect. Secondly, through its intracellular YVKM motif, CTLA-4 activates downstream inhibitory enzymes such as tyrosine phosphatase-2 and protein phosphatase-2A. Tyrosine phosphatase-2 dephosphorylates the CD3 chain, attenuating T cell receptor signaling, while protein phosphatase-2A dephosphorylates protein kinase B [28]. Yu conducted a study on 130 BC patients revealed CTLA-4 expression not only in BC cells but also in tumor-infiltrating lymphocytes. Patients with high total CTLA-4 expression exhibited poorer prognosis [29]. CTLA4 blockade can directly inhibit the proliferation of tumor cells expressing high levels of CTLA-4 and induce their apoptosis. Additionally, it alleviates dendritic cell inhibition, thereby restoring dendritic cell and CTL functions [30]. Ipilimumab, a monoclonal antibody that effectively blocks CTLA-4, was developed after decades [31]. The two main anti-CTLA-4 drugs used in BC research are ipilimumab and tremelimumab. CTLA-4 expression correlates with age in BC patients, with increased expression observed in older patients [32]. Dai suggested that CTLA4 + 6230G > A mutation could effectively reduce homozygous and recessive inheritance in the Chinese population, lowering the risk of BC [33]. High CTLA-4 expression corresponds to a low fraction of activated T cells, positively correlating with patient survival [34]. Furthermore, BC patients with higher CTLA-4 expression show a significant propensity for axillary lymph node metastasis [35].
CD39/CD73
CD39, an extracellular adenosine triphosphate hydrolase, and CD73, an extracellular nucleotidase, are ubiquitous in most tissues. CD39 catalyzes the hydrolysis of extracellular adenosine triphosphate and adenosine diphosphate to produce adenosine monophosphate, subsequently converted by CD73 into adenosine, which exhibits immunosuppressive properties. Adenosine binds to adenosine receptors on effector T cells and natural killer cells, elevating intracellular cyclic adenosine monophosphate levels. This leads to the upregulation of CD25, diminishing effector T cell proliferation, reducing immune factor production, and attenuating natural killer cell activity [36].
CD73 is expressed variably in normal mammary gland and BC tissues, with markedly increased expression and activity in metastatic BC. Its expression positively correlates with tumor grade and lymph node metastasis, while inversely correlating with estrogen receptor expression [37]. Allard reported that CD73 expression is higher in TNBC compared to other subtypes. This elevated expression associated with poorer prognosis and heightened resistance to chemotherapy drugs [38].
Transmembrane glycoprotein (LAG-3)
LAG-3 ligands, which are major histocompatibility complex class II (MHC-II) molecules, interact with LAG-3 to modulate T cell activity and dendritic cell differentiation [39]. This interaction can weaken T cell function and accelerate T cell failure. Additionally, LAG-3 indirectly inhibits T cell activity by regulating the function of CD4+ CD25+ Tregs [40] IMP321, a recombinant LAG3-Ig fusion protein, binds to MHC-II on activated antigen-presenting cells (APCs), activating memory CD8+ T cells and enhancing anti-tumor activity [41]. Expression of LAG-3 on immune cells has been observed in samples from patients with malignant pleural mesothelioma [42]. Galectin-3, expressed by CD8+ T cells, also contributes to the inhibition of anti-tumor responses [43]. Studies in primary non-small cell lung cancer have shown a correlation between LAG-3+ tumor-infiltrating lymphocytes (TILs) and disease-free survival. Co-administration of anti-LAG-3 antibody with anti-PD-1 antibody (nivolumab) has been found to enhance immune tolerance and promote T cell exhaustion, demonstrating efficacy in cancer treatment [44-45].
T cell Ig and mucin domain 3 (TIM-3)
IM-3 was initially identified as a specific marker of fully differentiated T cells secreting IFN-γ, including CD4+ Th1 and CD8+ cytotoxic T cells. Many studies have revealed that TIM-3 is also expressed on the surface of other immune cells, such as Tregs, mononuclear macrophages, and dendritic cells [46]. Its main mechanism of action involves the regulation of T cell proliferation and cytokine secretion. TIM-3 can exert its inhibitory effects by directly binding to CD4+ T cells, and it can also regulate the activity of CD4+ T cells when expressed on other immune cells [47]. Additionally, TIM-3 has been found to induce the expansion of bone marrow-derived suppressor cells [48]. CD8+ T cells are pivotal for tumor cell destruction, and TIM-3 released by tumors can impair their proliferation and effector function, enabling tumors to evade immune surveillance [49].
Indoleamine 2,3 dioxygenase (IDO)
IDO functions by depleting tryptophan levels within the tumor microenvironment, thereby directly impacting T cell activity. Additionally, its metabolite, kynurenine, has been shown to induce apoptosis in T cells. IDO may also contribute to immune tolerance by promoting the differentiation of T cells into regulatory T cells (TreGs) or by directly activating mature TreGs. Chen et al. [50] reported that IDO metabolites can enhance BC metastasis, with higher IDO expression correlating with poorer prognosis, particularly in the TNBC subgroup. Furthermore, Del has demonstrated the presence of IDO not only within BC cells but also in microvesicles within the BC microenvironment, suggesting its involvement in cancer cell migration and immune evasion [51].
B and T lymphocyte attenuator (BTLA)
BTLA, a co-inhibitory molecule with an immunoglobulin-like structure, is part of the CD28 family. Its cytoplasmic region contains ITIM and ITSM similar to CTLA-4 and PD-1, which exert negative regulation on peripheral immune tolerance and response. Upon binding to its ligand, BTLA exhibits negative immunomodulatory effects. While BTLA is highly expressed on B cells at rest, its expression on T cells, PDC cells, KT cells, and natural killer cells is relatively low. Upon activation, BTLA expression decreases in B cells but increases, particularly in Th1 cells, representing a reverse interaction [52]. Despite its role in maintaining T cell survival, BTLA restricts proliferation and activity, thereby promoting peripheral immune tolerance and constraining anti-tumor immunity. Moreover, BTLA can synergistically inhibit T cell function alongside other co-inhibitory molecules like PD-1 and TIM-3 [53], solidifying its status as a pivotal T cell inhibitory receptor.
TIGIT, a member of the immunoglobulin superfamily, possesses an immunoreceptor tyrosine-based inhibitory motif (ITIM) and a short intracellular domain phosphorylation motif akin to the immunoglobulin tyrosine tail (ITT) [54]. TIGIT inhibitors currently in development include Vibostolimab and Tiragolumab. Tirelliumab, the primary drug for HER2-high BC treatment, often encounters resistance due to inadequate antibody-dependent cellular cytotoxicity (ADCC) triggered by natural killer (NK) cells [55]. Xu investigated the potential of TIGIT blockade in enhancing the anti-tumor response of human NK cells activated by Trastuzumab. By incubating purified NK cells with Trastuzumab-coated BC cells and adding TIGIT inhibitors, significant elevation in interferon-γ (IFN-γ) production by NK cells was observed compared to controls [56]. These findings suggested that TIGIT blockade enhances cytokine secretion in NK cells upon stimulation by Trastuzumab-coated BC cells, augmenting anti-tumor activity. Tegaserod maleate (TM), a serotonin receptor 4 agonist, demonstrates promising therapeutic effects in BC [57].
VISTA
VISTA, a type I transmembrane protein, can bind to PSGL-1 on T cells at acidic pH, resulting in the inhibition of T cell function [58]. BC tissues exhibit higher VISTA expression levels compared to adjacent normal tissues, with VISTA being the most expressed immune checkpoint in BC tissues. VSTB112 inhibits VISTA's interaction with PSGL-1 and VSIG3. BMS-767 is the sole pH-selective monoclonal antibody, inhibiting the interaction between PSGL-1 and VSIG3 specifically at a low pH of 6.0.
Combined application of immune checkpoint suppression and chemotherapy
Traditionally, chemotherapy was thought to suppress the immune system, leading to belief that it cannot be combine with immunotherapy. However, recent studies suggest that immunotherapy can enhance tumor cells sensitivity to chemotherapy drugs. In metastatic breast cancer cell models, researchers observed that PD-1/PD-L1 pathway blockers can enhance the activity of doxorubicin in inhibiting tumor metastasis. Combining PD-1 blocked with doxorubicin significantly promote tumor regression and prolongs survival [60].
Combined application of immune checkpoint suppression and radiotherapy
Although radiotherapy is commonly associated with immunosuppressive effects, it can activate the immune system at appropriate doses. Radiotherapy induces the recruitment of activated cytotoxic T cells to the tumor microenvironment, facilitating tumor cell destruction and immune modulation [61]. Additionally, radiation therapy can trigger systemic reactions, known as ectopic effects, which synergize effectively with immunotherapy, such as immune checkpoint blockade or adoptive cell therapy [62]. Therefore, the judicious use of radiation not only targets tumor cells locally to curb tumor growth but also induces systemic ectopic effects to impede metastasis. When combined with immunotherapy, radiation therapy yields superior outcomes compared to monotherapy.
Combined application of immune checkpoint inhibition and tumor vaccine
Breast cancer, characterized by low immunogenicity, presents challenges for immunotherapy. However, the combination of tumor vaccines with immune checkpoint inhibitors offers promising avenues to address this limitation. Petrizzo explored the efficacy of a multiple peptide vaccine (PEPT) in combination with metronomic chemotherapy (MCT) and immune checkpoint inhibitors (CI). Results indicated that compared to PEPT alone, the combinations of PEPT+MCT and PEPT+CI demonstrated significant enhancements in tumor growth inhibition, survival rates, and reduction of regulatory T cells. Particularly, the combination of PEPT+MCT+CI yielded the most favorable outcomes in these aspects [63].
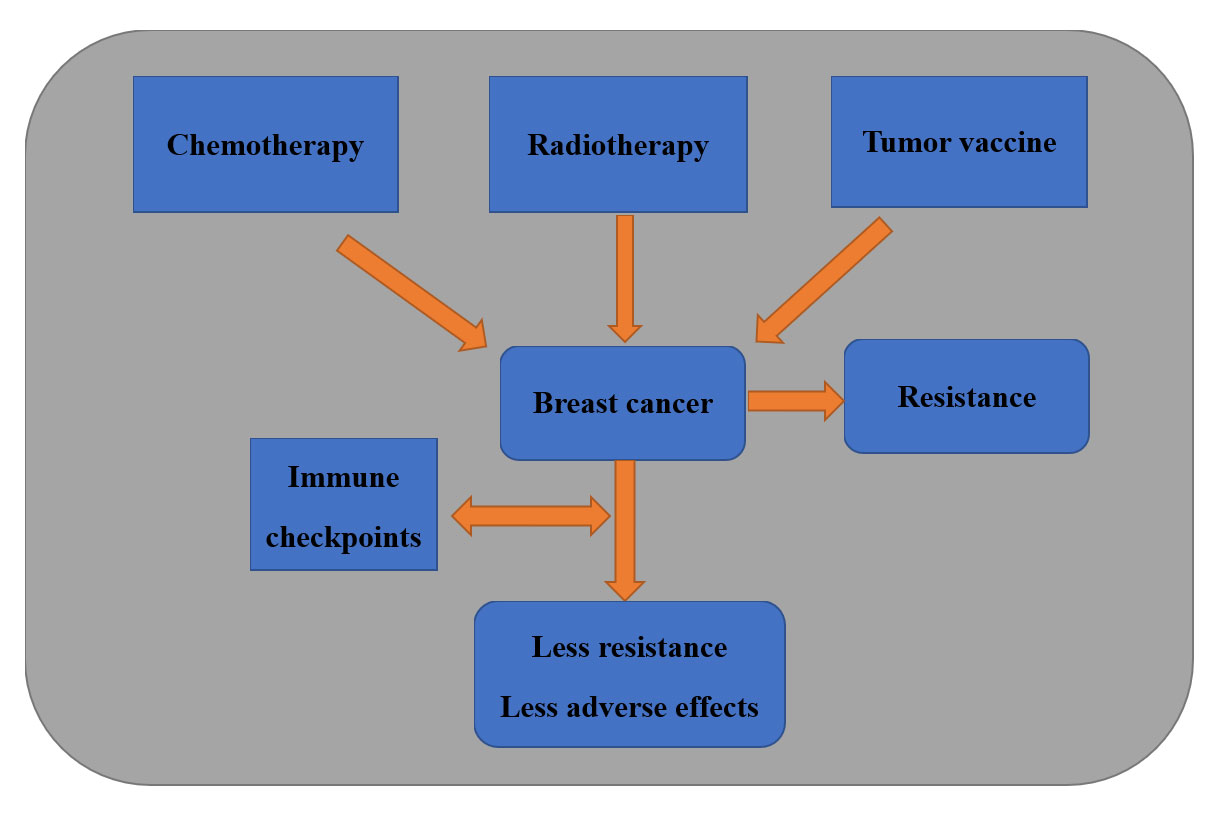
Despite significant progress, drug resistance to existing immune checkpoint inhibitors remains a common challenge and limiting patient outcomes. The current approach is to mitigate immune-related adverse reactions while optimizing immunotherapy efficacy. It can be achieved either by using combination strategies such as pairing immune checkpoint inhibitors with chemotherapy, radiotherapy, or targeted oncogene therapy have shown promise, positioning immunotherapy as a pivotal approach in breast cancer treatment. As novel targets emerge, the focus of future research extends to assessing the synergistic effects of various therapies to discover the best combinations yielding optimum clinical outcome. Additionally, there is a crucial need to investigate and identify relevant biomarkers capable of predicting tumor responsiveness to these treatment strategies, thereby augmenting their efficacy and precision.
None.
Availability of data and materials
Data and materials are available on request from the authors.
Ethical policy
Not applicable.
Author contributions
XYZ and JW conceptualized, designed, conducted research, and wrote the first draft; KYZ revised the draft.
Competing interests
The authors have no conflicts of interest regarding the publication of this paper.
Funding
None.
- Chen S, Mellman I: Elements of cancer immunity and the cancer-immune set point. Nature 2017, 541(7637): 321-330.
- Speiser E, Ho C, Verdeil G: Regulatory circuits of T cell function in cancer. Nat Rev Immunol 2016, 16(10): 599-611.
- Sahin U, Türeci Ö: Personalized vaccines for cancer immunotherapy. Science 2018, 359(6382): 1355-1360.
- Dieci V, Miglietta F, Guarneri V: Immune Infiltrates in Breast Cancer: Recent Updates and Clinical Implications. Cells 2021, 10(2): 223.
- Xia L, Oyang L, Lin J, Tan S, Han Y, Wu N, Yi P, Tang L, Pan Q, Rao S et al: The cancer metabolic reprogramming and immune response. Mol Cancer 2021, 20(1): 28.
- Li B, Chan L, Chen P: Immune Checkpoint Inhibitors: Basics and Challenges. Curr Med Chem 2019, 26(17): 3009-3025.
- Van der Vlist M, Kuball J, Radstake TR, Meyaard L: Immune checkpoints and rheumatic diseases: what can cancer immunotherapy teach us? Nat Rev Rheumatol 2016, 12(10): 593-604.
- Voorwerk L, Slagter M, Horlings HM, Sikorska K, van de Vijver KK, de Maaker M, Nederlof I, Kluin RJC, Warren S, Ong S et al: Immune induction strategies in metastatic triple-negative breast cancer to enhance the sensitivity to PD-1 blockade: the TONIC trial. Nat Med 2019, 25(6): 920-928.
- Wu SY, Xu Y, Chen L, Fan L, Ma XY, Zhao S, Song XQ, Hu X, Yang WT: Combined angiogenesis and PD- 1 inhibition for immunomodulatory TNBC: concept exploration and biomarker analysis in the FUTURE- CPlus trial. Mol Cancer 2022, 21(1): 84.
- Dong Y, Sun Q, Zhang X: PD-1 and its ligands are important immune checkpoints in cancer. Oncotarget 2017, 8(2): 2171-2186.
- Beldi-ferchiou A, Caillat-zucman S: Control of NK cell activation by immune checkpoint molecules. Int J Mol Sci 2017, 18(10): 2129.
- Kwapisz D: Pembrolizumab and atezolizumab in triple-negative breast cancer. Cancer Immunol Immunother 2021, 70(3): 607-617.
- Chen S, Mellman I: Elements of cancer immunity and the cancer-immune set point. Nature 2017, 541(7637): 321-330.
- Voorwerk L, Slagter M, Horlings HM, Sikorska K, van de Vijver KK, de Maaker M, Nederlof I, Kluin RJC, Warren S, Ong S et al: Immune induction strategies in metastatic triple-negative breast cancer to enhance the sensitivity to PD-1 blockade: the TONIC trial. Nat Med 2019, 25(6): 920-928.
- Wu SY, Xu Y, Chen L, Fan L, Ma XY, Zhao S, Song XQ, Hu X, Yang WT, Chai WJ et al: Combined angiogenesis and PD- 1 inhibition for immunomodulatory TNBC: concept exploration and biomarker analysis in the FUTURE- CPlus trial. Mol Cancer 2022, 21(1): 84.
- Kalbasi A, Ribas A: Tumour-intrinsic resistance to immune 484 ONCOLOGY PROGRESS, Mar 2023 Vol. 21 No. 5. checkpoint blockade. Nat Rev Immunol 2020, 20(1): 25-39.
- Shlapatska LM, Mikhalap SV, Berdova AG, Zelensky OM, Yun TJ, Nichols KE, Clark EA, Sidorenko SP et al: CD150 association with either the SH2-containing inositol phosphatase or the SH2- containing protein tyrosine phosphatase is regulated by the adaptor protein SH2D1A. J Immunol 2001, 166(9): 5480-5487.
- Nirschl J, Drake G: Molecular pathways: coexpression of immune checkpoint molecules: signaling pathways and implications for cancer immunotherapy. Clin Cancer Res 2013, 19(18): 4917-4924.
- Stovgaard ES, Dyhl-Polk A, Roslind A, Balslev E, Nielsen D: PD-L1 expression in breast cancer: expression in subtypes and prognostic significance: a systematic review. Breast Cancer Res Treat 2019, 174(3): 571-584.
- Bertucci F, Finetti P, Colpaert C, Mamessier E, Parizel M, Dirix L, Viens P, Birnbaum D, van Laere S: PDL1 expression in inflammatory breast cancer is frequent and predicts for the pathological response to chemotherap. Oncotarget 2015, 6(15): 13506-13519.
- Wimberly H, Brown JR, Schalper K, Haack H, Silver MR, Nixon C, Bossuyt V, Pusztai L, Lannin DR, Rimm DL: PD-L1 expression correlates with tumor-infiltrating lymphocytes and response to neoadjuvant chemotherapy in breast cancer. Cancer Immunol Res 2015, 3(4): 326-332.
- Qin T, Zeng YD, Qin G, Xu F, Lu JB, Fang WF, Xue C, Zhan JH, Zhang XK, Zheng QF et al: High PD-L1 expression was associated with poor prognosis in 870 Chinese patients with breast cancer. Oncotarget 2015, 6(32): 33972-33981.
- Schalper KA, Velcheti V, Carvajal D, Wimberly H, Brown J, Pusztai L, Rimm DL: In situ tumor PD-L1 mRNA expression is associated with increased TILs and better outcome in breast carcinomas. Clin Cancer Res 2014, 20(10): 2773-2782.
- Li S, Chen L, Jiang J: Role of programmed cell death ligand-1 expression on prognostic and overall survival of breast cancer: A systematic review and meta-analysis. Medicine (Baltimore) 2019, 98(16): e15201.
- Beckers RK, Selinger CI, Vilain R, Madore J, Wilmott JS, Harvey K, Holliday A, Cooper CL, Robbins E, Gillett D et al: Programmed death ligand 1 expression in triple-negative breast cancer is associated with tumor infiltrating lymphocytes and improved outcome. Histopathology 2016, 69(1): 25-34.
- Botti G, Collina F, Scognamiglio G, Rao F, Peluso V, De Cecio R, Piezzo M, Landi G, De Laurentiis M, Cantile M et al: Programmed death ligand 1 (PD-L1) tumor expression is associated with a better prognosis and diabetic disease in triple negative breast cancer patients. Int J Mol Sci 2017, 18(2): 459.
- Li X, Wetherilt CS, Krishnamurti U, Yang J, Ma Y, Styblo TM, Meisel JL, Peng L, Siddiqui MT, Cohen et al: Stromal PD-L1 expression is associated with better disease-free survival in triple-negative breast cancer. Am J Clin Pathol 2016, 146(4): 496-502.
- Yu H, Yang J, Jiao S, Li Y, Zhang W, Wang J: Cytotoxic T lymphocyte antigen 4 expression in human breast cancer: implications for prognosis. Cancer Immunol Immunother 2015, 64(7): 853-860.
- Soleimani A, Taghizadeh E, Shahsavari S, Amini Y, Rashidpour H, Azadian E, Jafari A, Parizadeh MR, Mashayekhi K, Soukhtanloo M et al: CD73, a key ectonucleotidase in the development of breast cancer: Recent advances and perspectives. Cell Physiol 2019, 234(9): 14622-14632.
- Chen X, Shao Q, Hao S, Zhao Z, Wang Y, Guo X, He Y, Gao W, Mao H: CTLA-4 positive breast cancer cells suppress dendritic cells maturation and function. Oncotarget 2017, 8(8): 13703-13715.
- Emens LA: Re-purposing cancer therapeutics for breast cancer immunotherapy. Cancer Immunol Immunother 2012, 61(8): 1299-1305.
- Kolacinska A, Cebula-Obrzut B, Pakula L, Chalubinska-Fendler J, Morawiec-Sztandera A, Pawlowska Z, Zawlik I, Morawiec Z, Jesionek-Kupnicka D, Smolewski P: Immune checkpoints: cytotoxic T-lymphocyte antigen 4 and programmed cell death protein 1 in breast cancer surgery . Oncol Lett 2015, 10(2): 1079-1086.
- Dai Z, Tian T, Wang M, Liu X, Lin S, Yang P, Liu K, Zheng Y, Xu P, Liu M et al: CTLA-4 polymorphisms associate with breast cancer susceptibility in Asians: a meta-analysis. Peer J 2017, 5: e2815.
- Lu L, Bai Y, Wang Z: Elevated T cell activation score is associated with improved survival of breast cancer. Breast Cancer Res Treat 2017, 164(3): 689-696.
- Mao H, Zhang L, Yang Y, Zuo W, Bi Y, Gao W, Deng B, Sun J, Shao Q, Qu X: New insights of CTLA-4 into its biological function in breast cancer. Curr Cancer Drug Targets 2010, 10(7): 728-736.
- Hasmim M, Berchem G, Janji B: A role for EMT in CD73 regulation in breast cancer. Oncoimmunology 2022, 11(1): 2152636.
- Allard B, Turcotte M, Stagg J: Targeting CD73 and downstream adenosine receptor signaling in triple-negative breast cancer. Expert Opin Ther Targets 2014, 18(8): 863-881.
- Hou Y, Nitta H, Wei L, Banks PM, Parwani AV, Li Z: Evaluation of immune reaction and PD-L1 expression using multiplex immunohistochemistry in HER2-positive breast cancer: the association with response to anti-HER2 neoadjuvant therapy. Clin Breast Cancer 2018, 18(2): e237-e244.
- Sasidharan Nair V, Elkord E: Immune checkpoint inhibitors in cancer therapy: a focus on T-regulatory cells. Immunol Cell Biol 2018, 96(1): 21-33.
- Fujio K, Yamamoto K, Okamura T: Overview of LAG-3-expressing, IL-10-producing regulatory T cells. Curr Top Microbiol Immunol 2017, 410: 29-45.
- Granier C, De Guillebon E, Blanc C, Roussel H, Badoual C, Colin E, Saldmann A, Gey A, Oudard S, Tartour E: Mechanisms of action and rationale for the use of checkpoint inhibitors in cancer. ESMO Open 2017, 2(2): e000213.
- Marcq E, Waele J, Audenaerde JV, Lion E, Santermans E, Hens N, Pauwels P, van Meerbeeck JP, Smits ELJ: Abundant expression of TIM-3, LAG-3, PD-1 and PD-L1 as immunotherapy checkpoint targets in effusions of mesothelioma patients. Oncotarget 2017, 8(52): 89722-89735.
- Granier C, De Guillebon E, Blanc C, Roussel H, Badoual C, Colin E, Saldmann A, Gey A, Oudard S, Tartour E: Mechanisms of action and rationale for the use of checkpoint inhibitors in cancer. ESMO Open 2017, 2(2): e000213.
- Hald SM, Rakaee M, Martinez I, Richardsen E, Al-Saad S, Paulsen EE, Blix ES, Kilvaer T, Andersen S, Busund LT et al: LAG-3 in non-small-cell lung cancer: expression in primary tumors and metastatic lymph nodes is associated with improved survival. Clin Lung Cancer 2018, 19(3): 249-259.
- Attili I, Passaro A, Pavan A, Conte P, De Marinis F, Bonanno L: Combination immunotherapy strategies in advanced non-small cell lung cancer (NSCLC): does biological rationale meet clinical needs? Crit Rev Oncol Hematol 2017, 119: 30-39.
- Hald SM, Rakaee M, Martinez I, Richardsen E, Al-Saad S, Paulsen EE, Blix ES, Kilvaer T, Andersen S, Busund LT et al: LAG-3 in non-small-cell lung cancer: expression in primary tumors and metastatic lymph nodes is associated with improved survival. Clin Lung Cancer 2018, 19(3): 249-259.
- Burugu S, Gao D, Leung S, Chia SK, Nielsen TO: LAG-3+ tumor infiltrating lymphocytes in breast cancer: clinical correlates and association with PD-1/PD-L1+ tumors. Ann Oncol 2017, 28(12): 2977-2984.
- Bottai G, Raschioni C, Losurdo A, Di Tommaso L, Tinterri C, Torrisi R, Reis-Filho JS, Roncalli M, Sotiriou C, Santoro A et al: An immune stratification reveals a subset of PD-1/LAG-3 double-positive triple-negative breast cancers. Breast Cancer Res 2016, 18(1): 121.
- Lee YH, Martin-Orozco N, Zheng P, Li J, Zhang P, Tan H, Park HJ, Jeong M, Chang SH, Kim BS, Xiong W et al: Inhibition of the B7-H3 immune checkpoint limits tumor growth by enhancing cytotoxic lymphocyte function. Cell Res 2017, 27(8): 1034-1045.
- Obeid M, Tesniere A, Ghiringhelli F, Fimia GM, Apetoh L, Perfettini JL, Castedo M, Mignot G, Panaretakis T, Casares N et al: Calreticulin exposure dictates the immunogenicity of cancer cell death. Nat Med 2007, 13(1): 54-61.
- Del Rio L, Kaye J, Rodriguez-Barbosa I: Detection of protein on BTLAlow cells and in vivo antibody-mediated down-modulation of BTLA on lymphoid and myeloid cells of C57BL/6 and BALB/c BTLA allelic variants. Immunobiology 2010, 215(7): 570-578.
- Fourcade J, Sun Z, Pagliano O, Guillaume P, Luescher F, Sander C, Kirkwood M, Olive D, Kuchroo V, Zarour M: CD8(+) T cells specific for tumor antigens can be rendered dysfunctional by the tumor microenvironment through upregulation of the inhibitory receptors BTLA and PD-1. Cancer Res 2012, 72(4): 887-896.
- Harjunpää H, Guillerey C: TIGIT as an emerging immune checkpoint. Clin Exp Immunol 2020, 200(2): 108-119.
- Chauvin M, Zarour M: TIGIT in cancer immunotherapy. Immunother Cancer 2020, 8(2): e000957.
- Xu F, Sunderland A, Zhou Y, Schulick D, Edil H, Zhu Y: Blockade of CD112R and TIGIT signaling sensitizes human natural killer cell functions. Cancer Immunol Immunother 2017, 66(10): 1367-1375.
- Guo C, Luo Z, Ismtula D, Bi X, Kong H, Wang Y, Yang Z, Mao X: TIGIT is a novel prognostic marker and correlate for immune infiltration in invasive breast cancer. Comb Chem High Throughput Screen 2022, 20(1): 25-39.
- Wang J, Wu G, Manick B, Hernandez V, Renelt M, Erickson C, Guan J, Singh R, Rollins S, Solorz A et al: VSIG- 3 as a ligand of VISTA inhibits human T- cell function. Immunology 2019, 156(1): 74-85.
- Sanchez K, Page D, Mcarthur L: Immunotherapy in breast cancer: an overview of modern checkpoint blockade strategies and vaccines. Curr Probl Cancer 2016, 40(2-4): 151-162.
- Terranova-Barberio M, Thomas S, Ali N, Pawlowska N, Park J, Krings G, Rosenblum MD, Budillon A, Munster PN: HDAC inhibition potentiates immunotherapy in triple negative breast cancer. Oncotarget 2017, 8(69): 114156-114172.
- Hu I, Ho Y, Mcarthur L: Combined radiation therapy and immune checkpoint blockade therapy for breast cancer. Int J Radiat Oncol Biol Phys 2017, 99(1): 153-156.
- Weichselbaum R, Liang H, Deng L, Fu X: Radiotherapy and immunotherapy: a beneficial liaison? Nat Rev Clin Oncol 2017, 14(6): 365-379.
- Esposito A, Criscitiello C, Curigliano G: Immune checkpoint inhibitors with radiotherapy and locoregional treatment: synergism and potential clinical implications. Curr Opin Oncol 2015, 27(6): 445-451.
- Petrizzo A, Mauriello A, Luciano A, Rea D, Barbieri A, Arra C, Maiolino P, Tornesello M, Gigantino V, Botti G et al: Inhibition of tumor growth by cancer vaccine combined with metronomic chemotherapy and anti-PD-1 in a pre-clinical setting. Oncotarget 2018, 9(3): 3576-3589.
Asia-Pacific Journal of Oncology
print ISSN: 2708-7980, online ISSN: 2708-7999
Copyright © Asia Pac J Oncol. This work is licensed under a Creative Commons Attribution-NonCommercial-No Derivatives 4.0 International (CC BY-NC-ND 4.0) License.