Review | Open Access
Research advances in tumor diagnosis and early detection
Rodney Bradly1
1Department of Natural Sciences, Shawnee State University, 940 Second Street, Portsmouth, OH 45662, USA.
Correspondence: Rodney Bradly (Department of Natural Sciences, Shawnee State University, 940 Second Street, Portsmouth, OH 45662, USA; Email: rodney_bradly86@zohomail.com).
Asia-Pacific Journal of Oncology 2024, 5: 46-54. https://doi.org/10.32948/ajo.2024.09.18
Received: 13 Sep 2024 | Accepted: 21 Sep 2024 | Published online: 08 Oct 2024
This review explores recent advances in tumor diagnosis and early detection, focusing on cutting-edge developments in molecular diagnostic technologies, imaging techniques, and the integration of multi-omics data. Current tumor diagnostic methods have limitations in terms of sensitivity and specificity, particularly for early tumor detection. However, with continuous progress in research and emerging technologies, especially the advent of liquid biopsy, which enables the detection of circulating tumor DNA (ctDNA), exosomes, and tumor-educated platelets (TEPs), the sensitivity and accuracy of early cancer detection have significantly improved. Moreover, the combined application of artificial intelligence and high-resolution imaging technology has enhanced the precision of diagnosis. Despite these advances, challenges, such as the high cost of technology and difficulties in data integration, continue to impede widespread clinical adoption. Therefore, I believe that future research should prioritize the innovation of these technologies to improve their applicability for early detection across various cancer types, ultimately contributing to the advancement of personalized therapy.
Key words early cancer detection, molecular diagnostics, liquid biopsy, exosomes, personalized treatment
Gradually, the use of liquid biopsy has enhanced early cancer detection, particularly for detecting circulating tumor DNA (ctDNA) [4]. For example, this technique has been applied in the early detection of pancreatic ductal adenocarcinoma (PDAC) [5]. Precision medicine can benefit greatly from liquid biopsies as it can also be used to monitor treatment response and detect minimal residual disease. By identifying small amounts of tumor-related gene fragments in the blood, cancer can be detected at an early, often asymptomatic, stage, allowing for early intervention [6].
In recent years, there has been a growing exploration of integrating molecular imaging technologies with advancements in artificial intelligence. The current literature demonstrates that combining these two strategies can enhance the identification of tumor characteristics and improve the sensitivity and specificity of detection [7]. Additionally, recent research has highlighted the potential of integrating genomic, proteomic, and epigenomic data to develop new methods for the early identification of cancer [8-10].
These developments lay the foundation for creating individualized treatment plans, while also offering new approaches and strategies for early cancer diagnosis. However, several challenges must still be addressed before these technologies can be widely used in clinical settings. These challenges include the need for technical standardization, the high costs associated with these solutions, and difficulties in integrating data from various methodologies [11]. Therefore, future research should focus on optimizing and advancing these techniques and exploring their applicability across different types of cancer to achieve broader and more effective early detection. This review aims to provide an overview of the latest advancements in tumor diagnosis and early detection. It also discusses the potential applications of emerging molecular diagnostic techniques, imaging technologies, and multi-omics integration strategies, weighing the benefits and drawbacks of these approaches in clinical settings, while speculating on future directions.
At this stage, we primarily rely on imaging techniques, such as X-rays, CT scans, magnetic resonance imaging, and histopathology, to examine or diagnose tumors. Although these methods are widely recognized and used by physicians in clinical practice, they have significant limitations and shortcomings. For example, imaging often detects tumors only after they have reached a certain size, and histopathology requires invasive procedures like biopsies [12, 13], which can lead to complications and make it more difficult to detect early, small, or dispersed tumor lesions. Furthermore, conventional assays provide limited information about the molecular characteristics of tumors and are often ineffective for personalized treatment. These limitations have driven the development of more sensitive and accurate diagnostic assays.
The development of tumor biomarkers
After years of investigation, some progress has finally been made in the study of tumor biomarkers. For example, traditional serum markers such as carcinoembryonic antigen (CEA) and prostate-specific antigen (PSA) are commonly used for tumor screening and monitoring. However, their specificity and sensitivity are limited, often resulting in false-positive or false-negative outcomes that complicate tumor detection [14, 15]. To enhance assay accuracy, researchers are developing more specific diagnostic techniques and identifying stable novel biomarkers. Among these, circulating tumor DNA (ctDNA) [16], microRNA (miRNA) [17], and exosomes [18] are considered promising categories of novel biomarker assays.
Through a thorough investigation of breast cancer, Itani et al. demonstrated the potential of miRNAs as biomarkers for early breast cancer diagnosis [17]. Similarly, Yu et al. reported the potential of exosomes as diagnostic markers of cancer [18]. Furthermore, another study illustrated additional uses of exosomes. Lu et al. discovered that exosomes released by macrophages might facilitate the spread of hepatocellular carcinoma, further highlighting the critical role exosomes play in cancer progression [18, 19]. In conclusion, these novel biomarkers can be detected using non-invasive techniques, such as liquid biopsy while providing crucial information about the molecular characteristics of tumors. This information can help to tailor early treatment interventions and establish a foundation for the prevention and treatment of early tumorigenesis.
(1) Exosomes as Biomarkers. Liquid biopsy is becoming a major noninvasive diagnostic method due to ongoing advancements in early cancer detection technologies. In addition to the well-researched circulating tumor cells (CTCs) and circulating tumor DNA (ctDNA), a diverse group of experts have also recognized exosomes and tumor-educated platelets (TEPs) as innovative biomarkers with significant potential for early cancer identification. As the study of these two biomarkers introduces new detection targets into the liquid biopsy technique, it is expected to further enhance the sensitivity and specificity of early cancer detection in the future. Exosomes are vesicles released by cells that can be detected in blood, urine, and saliva, with diameters typically ranging from 30 to 150 nanometers. They are capable of carrying a variety of biomolecules including DNA, RNA, proteins, and lipids. By detecting these biomolecules, it is possible to assess the state of the cell. Studies have shown that, in the tumor microenvironment, exosomes secreted by tumor cells are important carriers of cancer-associated molecules, reflecting tumor development. Exosomes from tumors have been reported to promote cancer cell proliferation, invasion, and metastasis by delivering regulatory molecules such as miRNAs, mRNAs, and proteins [19]. Therefore, exosomes are not only key biomarkers for early cancer diagnosis but also play a significant role in cancer progression. For instance, Tan and colleagues found a strong correlation between miRNAs and malignancies, showing that specific miRNAs were significantly expressed in exosomes secreted by malignant cells [20]. Ongoing research has further demonstrated a strong association between exosome-borne macromolecules and malignancies such as pancreatic, breast, and lung cancers. These exosomes, loaded with proteins, miRNAs, and other compounds, have been linked to numerous types of cancer. Additionally, the identification and extraction of the macromolecules carried by exosomes can be achieved through non-invasive assays, facilitating the early detection and diagnosis of cancer. Compared to ctDNA, exosomes are more stable in circulation and are secreted in relatively larger amounts, making them easier to detect. Moreover, according to a study by Tan et al., exosomes not only reflect the characteristics of tumor cells but also reveal alterations in the tumor microenvironment [21]. Analyzing exosome content, particularly miRNAs, proteins, and lipids, can provide crucial information on tumor status, disease progression, and potential treatment targets. Recent advancements in exosome detection and separation technologies, such as immunocapture and microfluidic methods, have further enhanced the potential of exosomes for clinical use as indicators of early cancer.
(2) Tumor-educated platelets (TEPs). First, platelets are not only essential for the coagulation process but also play a critical role in tumor development by interacting with the surrounding tissue. Recent studies, such as that by Best et al., have shown that platelets undergo a process known as tumor education, where they absorb molecular signals from tumors, leading to alterations in their gene expression [22]. These tumor-educated platelets (TEPs) carry tumor-derived RNA and proteins, reflecting the tumor's condition and contributing to disease progression [22]. TEPs have shown significant promise as novel cancer biomarkers, particularly in the early diagnosis of cancer. Tumor-educated platelets (TEPs) are formed through the secretion of regulatory chemicals such as chemokines and cytokines by tumor cells, which alter the platelet transcriptome. These transcriptome changes reflect the presence and characteristics of cancers. TEPs have been shown to distinguish between cancer patients and healthy individuals across various cancer types. RNA sequencing can identify cancer-specific gene expression profiles unique to different cancers [23]. Specifically, RNA sequencing of TEPs has been used to detect cancer-specific gene expression in various types of cancer. For example, patients with metastatic lung cancer exhibited significantly different RNA profiles compared to healthy individuals. In 2010, Calverley et al. discovered more than 200 differentially expressed RNAs [24]. Scientific studies have shown that analyzing the RNA content of platelets can reveal variations in the expression of cancer-related genes, aiding in the early detection of the disease. TEP detection is a noninvasive and highly sensitive method that can be used to identify various types of cancer. Recent research suggests that TEP RNA expression profiles can be used to detect early stage cancer and distinguish between different cancer types, including glioblastoma, lung cancer, and pancreatic cancer [25].
With the increasing use of next-generation sequencing (NGS), recent advances in genomic technologies have made precision medicine and early tumor detection more feasible [26]. NGS can identify various genetic mutations, copy number variations, gene fusions, and other abnormalities [27]. This information plays a critical role in early diagnosis and in guiding cancer treatment [28]. For example, genomic approaches are commonly used to detect EGFR mutations in lung cancer and BRAF mutations in melanoma, which are subsequently used for targeted therapy [29].
In 2002, Davies et al. pioneered the identification of the significance of BRAF mutations in cancer, particularly melanoma [30]. This discovery laid the foundation for subsequent studies on the BRAF V600E mutation, which has become a key target in melanoma therapies.
Furthermore, the extensive application of emerging fields, such as spatial transcriptomics and proteomics, has enhanced our understanding of tumor heterogeneity. By identifying genetic characteristics within the tumor microenvironment, these fields allow for more precise tumor classification and guide informed therapeutic decisions [31].
Epigenetic biomarkers
Epigenetic biomarkers have recently garnered significant attention in the field of early cancer detection. Epigenetics include processes such as DNA methylation, histone modifications, chromatin remodeling, and non-coding RNA regulation, which control gene expression without altering the DNA sequence. Epigenetic changes have been shown to be closely linked to the development and progression of various cancers, most notably aberrant DNA methylation in the promoter regions of tumor-associated genes. Jones and Baylin demonstrated that this abnormal DNA methylation plays a crucial role in cancer development and progression [32, 33]. These epigenetic changes often occur in the early stages of cancer, making them valuable tools for early detection [34].
Epigenetic markers such as DNA methylation and histone modifications are gaining increasing attention for the early detection of cancer. DNA methylation can affect normal cellular function by inhibiting gene expression. In cancer, the promoter regions of certain tumor suppressor genes frequently undergo aberrant methylation, leading to gene silencing and the promotion of tumor initiation and progression. Hu S and Shen Y et al. have shown that alterations in DNA methylation patterns, particularly aberrant methylation in the promoter regions of tumor-associated genes, are closely linked to the initiation and progression of various cancers [35, 36]. Additionally, the detection of methylation in the Septin9 gene is already being used for early colorectal cancer screening.
In addition to colorectal cancer, specific DNA methylation biomarkers have also been identified in other cancer types. For example, Oubaddou found that promoter methylation of the BRCA1 gene is closely associated with the development of breast cancer [37], whereas methylation of the RASSF1A and MGMT genes is commonly observed in lung cancer and glioblastoma [37]. Moreover, DNA methylation biomarkers have been used to detect endometrial, prostate, and liver cancer. These biomarkers can help to differentiate between precancerous and normal tissues and aid in early diagnosis. By utilizing these biomarkers, early lesions can be detected before cancer develops into significant foci, enabling timely intervention [38, 39].
Histone modification is another critical epigenetic alteration, which includes acetylation, methylation, phosphorylation, and ubiquitination. These modifications regulate gene expression by altering the chromatin structure. In tumors, abnormal histone modifications can lead to gene silencing or activation, driving cancer initiation and progression. In various cancers Modifications, such as trimethylation of histone H3 at lysine 27 (H3K27me3) and acetylation of histone H3 at lysine 9 (H3K9ac), have been associated with the inactivation of tumor suppressor genes. Zhou M et al. demonstrated that H3K27me3 enrichment led to the silencing of the SFRP1 gene, promoting cancer cell proliferation via the Wnt/β-catenin signaling pathway [40]. Additionally, Monaghan highlighted the emerging role of H3K9me3 as a potential therapeutic target in acute myeloid leukemia [41]. These histone modifications result in chromatin condensation, thereby repressing the expression of critical tumor suppressor genes [42]. For example, the abnormal silencing associated with H3K27me3 is closely linked to breast, prostate, and colorectal cancers [43]. Although histone modification detection techniques have not yet been widely applied in clinical settings, they continue to attract significant interest from researchers as potential cancer biomarkers.
Liquid biopsy
As a noninvasive technique, liquid biopsy offers a revolutionary tool for the early diagnosis of cancer by detecting circulating tumor DNA (ctDNA), circulating tumor cells (CTCs), and exosomes in the blood. Compared to traditional tissue biopsies, liquid biopsy not only allows for real-time monitoring of tumor dynamics but also captures tumor heterogeneity and evolutionary characteristics [44]. This capability provides liquid biopsies with significant potential for the early detection of multiple cancer types, including lung, breast, and colorectal cancers (Figure 1).
DNA fragments with genetic and epigenetic information, such as methylation and mutations unique to a tumor which are discharged into the bloodstream by tumor cells are known as circulating tumor DNA (ctDNA). Early cancer identification is made possible by the detection of ctDNA, which identifies specific tumor gene mutations, copy number variations and methylation anomalies. When it comes to early stages when the tumor burden is modest, which ctDNA testing has a higher sensitivity than tissue biopsy and can identify even minute genetic abnormalities [45]. In recent years, research on the application of ctDNA in cancers, such as lung cancer, colorectal cancer, and breast cancer, has intensified, with its sensitivity and specificity gradually improving [46].
Circulating tumor cells (CTCs) are tumor cells shed from the primary tumor or metastatic sites into the bloodstream. Since CTCs directly reflect tumor heterogeneity and invasiveness, their detection can be used not only for early cancer screening, but also for assessing the metastatic potential of tumors. Current CTC detection methods primarily involve separation techniques based on physical properties (such as size and density) and molecular markers. Although the detection rate of CTCs remains relatively low, this method is gaining increasing attention in clinical research as a valuable tool for early cancer diagnosis and metastatic risk prediction.
Liquid biopsy has shown promising results for the early detection of various cancers. For instance, Liu et al. found that EGFR mutations in lung cancer, KRAS mutations in colorectal cancer, and PIK3CA mutations in breast cancer could be identified early through ctDNA testing [47]. Multiplex analysis techniques in liquid biopsy allow for the integration of information from different biomarkers [46]. By combining ctDNA with other biomarkers such as proteins and RNA found in exosomes, a multidimensional molecular profile of cancer can be created, further enhancing the sensitivity and specificity of detection.
The main benefit of liquid biopsy is that it is non-invasive. Compared with tissue biopsies, which require surgical or needle-based procedures, liquid biopsies involve only the simple collection of blood or body fluids, minimizing patient discomfort and risk. Furthermore, liquid biopsy allows for the dynamic tracking of tumor growth, identifying potential drug resistance or gene alterations during therapy, and providing real-time data for adjusting treatment plans. Recently, there has been growing emphasis in liquid biopsy research on the development of novel techniques to improve the specificity and sensitivity of detection. Using multiplex analytic approaches to combine ctDNA with other biomarkers provides a more comprehensive representation of the genetic characteristics of the tumor, increasing detection accuracy and clinical value.
Tumor heterogeneity refers to the differences in biological behavior, gene expression, and mutation patterns among various populations of tumor cells. This concept is critical for early cancer detection and for targeted therapy.
Multi-omics integration and precision medicine
Multiomics integration is emerging as a major avenue for the molecular diagnosis of cancer. By combining data from various omics approaches, such as proteomics, genomics, and epigenomics, the sensitivity and specificity of tumor detection can be enhanced through a more comprehensive understanding of their biological features. This combined strategy is valuable not only for early identification but also for providing crucial molecular data that can guide the development of individualized treatment plans. For example, integrated data can help identify specific tumor subtypes, which may aid in the development of targeted therapies tailored to these subtypes [48].
Proteomics and metabolomics have shown that tumor cells undergo dynamic changes at the protein and metabolite levels. These changes often result from genetic mutations and epigenetic alterations. Combined proteomic and metabolomic data offer researchers a deeper understanding of the biological activities of tumors. For instance, Li et al. discovered that the reprogramming of metabolic pathways in cancer cells is a crucial marker of tumor growth. Abnormal metabolic activity in tumor cells, as revealed by metabolic data, enables detection even at the stage of microlesion formation [49]. Integration of genomics and epigenomics can also detect alterations in DNA methylation and gene mutations. Furthermore, Sun et al. found that abnormal methylation of the promoter regions of certain tumor suppressor genes occurs early in cancer development. When combined with information on genetic mutations, these anomalies can be used for early cancer detection [50].
For instance, Shen et al. found that the accuracy of early cancer diagnosis, such as for colorectal, lung, and breast cancers, improved by combining DNA methylation and mutation data [51]. Their research demonstrated that the sensitivity and specificity of early cancer screening can be enhanced by using a combination of gene mutations and DNA methylation patterns to distinguish cancer cells from normal cells. Additionally, studies have revealed a strong correlation between specific methylation markers and certain cancer subtypes, offering new perspectives for personalized cancer care. By integrating multi-omics data, different molecular subtypes of cancers can be identified, as different tumor subtypes may exhibit distinct biological behaviors and drug sensitivities. For example, Golestan et al. and Lee et al. discovered that combining gene expression profiles with epigenetic features in breast cancer can distinguish subtypes such as HER2-positive and hormone receptor-positive cancers [51, 52]. Their findings demonstrated that these subtypes possess unique molecular signatures that influence their responses to targeted therapies. After identifying the tumor subtypes, medical professionals can create individualized targeted treatment plans based on the unique characteristics of each patient's tumor.
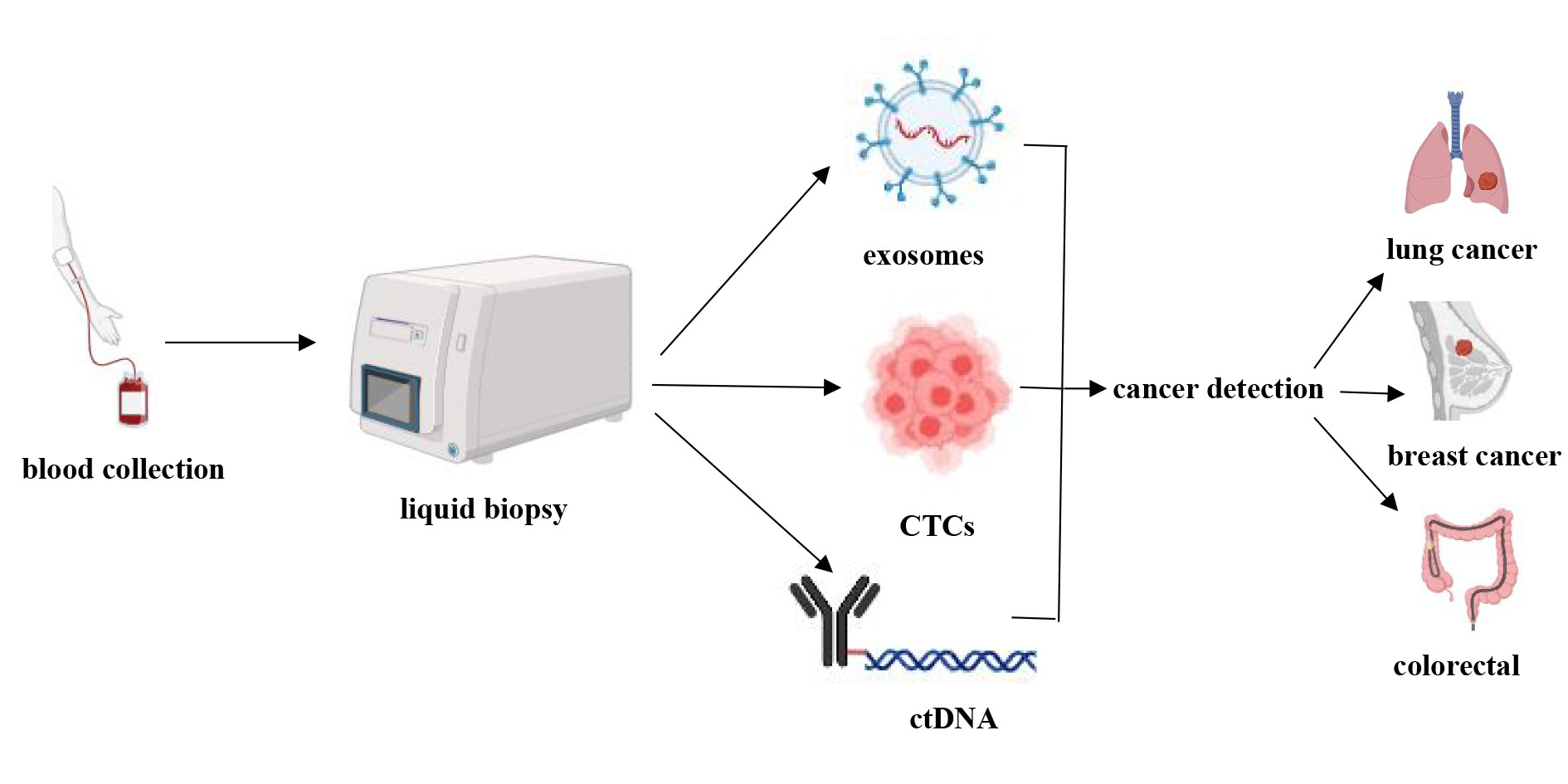
In recent years, notable advancements have been made in imaging technologies for early cancer detection. High-resolution images, which are essential for detecting small tumor lesions, have been obtained using conventional MRI and CT techniques. For instance, high-field MRI and advanced CT technologies offer a more detailed view of the structural properties of tumor tissues, thereby improving the accuracy of early cancer diagnosis [53]. By combining PET/CT, researchers can simultaneously obtain metabolic and anatomical data, allowing for more precise cancer staging and the evaluation of treatment effectiveness.
Magnetic resonance imaging (MRI) is a common non-invasive imaging technique used for early cancer detection. While traditional MRI provides clear soft-tissue contrast images, high-field MRI has recently been introduced to further enhance the image quality and clarity. With a field strength of 3T (Tesla) or greater and a higher signal-to-noise ratio (SNR), high-field MRI improves the detection of small lesions. This technology is particularly valuable in the early screening of brain, prostate, and breast cancers, as it reveals the fine structures of tumor tissues in more detail, aiding in the early identification of potential lesions.
Traditional computed tomography (CT) uses X-rays to produce cross-sectional anatomical images. Recently, advanced CT technology has enhanced the accuracy of this diagnostic tool for early cancer detection. Enhanced CT, which uses an intravenous contrast injection to significantly improve the contrast between the tumor and normal tissues, is particularly useful for detecting early malignancies in the liver, pancreas, and lungs. High-resolution computed tomography (CT) not only helps identify small tumors, but also provides precise images of tumor boundaries and morphological features, offering critical information for evaluating tumor characteristics and developing appropriate treatment plans.
While CT accurately localizes the tumor anatomically, PET scans reflect the metabolic activity of tumor cells by detecting the uptake of radioactive tracers such as 18F-FDG. The integration of PET and CT allows doctors to view both the tumor's structural characteristics and metabolic changes simultaneously, enabling a comprehensive evaluation. For example, Li et al. found that PET/CT can effectively differentiate between benign and malignant lesions in malignancies with high metabolic activity, such as lymphoma and lung cancer, and can assist in detecting early lesions that are difficult to identify using traditional imaging methods [54].
Mallum et al. found that PET/CT not only aids in the early detection of cancer but also plays a crucial role in tumor staging and treatment evaluation [55]. Their study demonstrated that PET/CT is highly effective in monitoring the response to cancer therapies such as radiotherapy and chemotherapy by tracking dynamic changes in tumor metabolic activity. A reduction in metabolic activity after treatment can serve as a marker of therapeutic success, whereas persistently active metabolic regions may indicate residual tumors or risk of recurrence. Additionally, PET/CT is important for detecting cancer metastasis because it can identify small metastatic lesions that are difficult to detect using other imaging techniques.
Molecular imaging technology
Tumors can be imaged at the molecular level using molecular imaging technologies and specific contrast agents. Advancements in this technology allow not only the detection of tumors but also the assessment of their molecular characteristics, such as metabolic activity and receptor expression. According to a recent study by Gao et al., HER2 receptor-based PET imaging agents show great promise for detecting and monitoring HER2-positive breast cancer [56]. This type of molecular imaging technology enables individualized early cancer detection and contributes to the development of more precise treatment plans for patients.
Molecular imaging technology is primarily applied in receptor-targeted imaging, such as HER2 in breast cancer and PSMA in prostate cancer, which are frequently expressed on the surface of tumor cells. By utilizing molecular probes that exploit the specific expression of these receptors, targeted imaging can be performed. For instance, Yeh et al. demonstrated the significant potential of PET imaging agents targeting the HER2 receptor for detecting and monitoring HER2-positive breast cancer [57]. These probes can bind to tumor cells with strong HER2 receptor expression, thus providing clear tumor imaging. This technology not only allows for the accurate detection of tumor presence but also assesses receptor expression levels, offering valuable data for developing individualized treatment plans. An abnormally high metabolic activity is a hallmark of tumor cells. By identifying metabolic processes in tumor cells, such as glucose and amino acid metabolism, molecular imaging techniques can be used to visualize tumors. In PET imaging, fluorodeoxyglucose (FDG) takes advantage of the high glucose uptake by tumor cells to provide metabolic imaging of malignancies. This method has been widely used for the postoperative surveillance and early detection of various cancers, including lymphoma, colorectal cancer, and lung cancer [58, 59]. Additionally, amino acid metabolism imaging has shown significant advantages in the early detection of neurological tumors. Molecular imaging technology has broad potential for the early detection, diagnosis, and monitoring of cancer [60]. Using molecular probes that bind to specific tumor biomarkers, small tumor lesions, particularly in the early stages of cancer, can be identified, which may not be detectable by traditional imaging techniques. This capability is crucial for improving the cure rates and overall prognosis.
Artificial intelligence and radiomics
The integration of artificial intelligence (AI) and radiomics has revolutionized imaging analyses. Through deep learning algorithms, AI can automatically extract and analyze key features from large volumes of imaging data. This not only accelerates the diagnostic process but also significantly enhances the sensitivity and specificity of detection. By performing high-throughput feature extraction from imaging data, radiomics can reveal intratumoral heterogeneity, aiding in the identification of early cancer characteristics.
The application of these technologies has demonstrated notable advantages in the early detection of various cancers, including brain tumors [61-64], lung cancer [65, 66], and breast cancer [67-69]. Several recent studies have highlighted the potential for certain applications (Table 1).
Multimodal imaging technology
Current research focuses on the integration of multimodal imaging technology, which combines several imaging modalities, such as MRI-CT and PET-CT, to provide more detailed information about tumors. Roberto et al. discovered that combining structural, functional, and molecular imaging enhanced the accuracy of malignant lesion identification, significantly increasing the success rate of early detection [70]. By combining multiple imaging modalities, a comprehensive understanding of the molecular markers, metabolic activity, and physical characteristics of the tumor can be obtained. Multimodal imaging is also crucial for the dynamic monitoring of tumor treatment, allowing for the rapid assessment of treatment effectiveness.
There are clear benefits to using multimodal imaging technology for early cancer detection. The sensitivity and resolution limitations of individual imaging modalities can make it challenging to detect small tumors at an early stage. However, by merging the information from multiple modalities, multimodal imaging can increase the likelihood of successful early detection. Hofman et al. found that PET-CT can simultaneously identify anatomical abnormalities and metabolic activity in malignancies [71]. It has proven to be sensitive in detecting cancers, including lung, breast, and colorectal cancers. The combination of metabolic and structural imaging with PET-CT allows for a more accurate tumor diagnosis, particularly in the early stages when other imaging modalities may miss malignant lesions [72].
Furthermore, comprehensive imaging can identify microtumors or precancerous lesions at an earlier stage, allowing for earlier diagnosis and treatment strategies based on molecular markers. Assessing the location and size of tumors is crucial for cancer staging. This imaging technique provides physicians with a complete evaluation of the tumor's invasion depth, lymph node involvement, and distant metastases by combining high-precision anatomical imaging with functional tumor data. A thorough assessment directly affects accurate staging and treatment decisions, helping medical professionals make the best choices and predict patient outcomes. In addition to its importance in early detection, multimodal imaging technology is also valuable for dynamic monitoring during therapy. Kim N et al. showed that PET-CT can measure real-time tumor metabolic changes during chemotherapy or radiation therapy, allowing for the assessment of treatment effectiveness [73].
Their study showed that changes in metabolic absorption, such as decreases in the maximum standardized uptake value (SUVmax) of tumors, are strongly correlated with treatment progress and can be identified during therapy. This real-time evaluation helps minimize medication side effects, avoids ineffective therapies, and allows for early adjustments to treatment regimens. By utilizing comparative imaging methods, clinicians can track changes in tumor size and angiogenesis, providing deeper insights into treatment effectiveness. Cancer treatment often requires timely adjustments based on dynamic tumor changes, and the multidimensional information provided by multimodal imaging supports these adjustments. By tracking metabolic activity, blood flow, and structural alterations in tumors, clinicians can assess whether the tumor responds to treatment and make the necessary changes if resistance or recurrence is observed. For patients undergoing targeted immunotherapy, multimodal imaging can capture molecular and metabolic changes in the tumor, helping to identify treatment effectiveness or reasons for failure.
Table 1. The application of artificial intelligence in the study of influence. |
||||
Cancer type |
Imaging technology |
AI model |
Key findings |
Reference |
Brain tumor |
MRI |
Neural networks, transfer learning |
Improved MRI-based classification accuracy for brain tumors |
[61] |
Lung cancer |
CT |
Deep learning |
Hybrid deep learning model outperforms traditional methods in lung cancer detection |
[65] |
Breast cancer |
Digital mammography |
Transfer learning |
High efficiency in breast cancer detection with reduced false positive rates |
[67] |
Brain tumor |
MRI |
Ensemble learning, K-Nearest neighbors |
High accuracy and robustness in brain tumor detection |
[62] |
Brain tumor |
MRI |
Neural networks, transfer learning |
Improved MRI-based classification accuracy for brain tumors |
[63] |
Lung cancer |
CT |
Deep learning |
Hybrid deep learning model outperforms traditional methods in lung cancer detection |
[66] |
Breast cancer |
Digital mammography |
Transfer learning |
High efficiency in breast cancer detection with reduced false positive rates |
[68] |
Brain tumor |
MRI |
Ensemble learning, K-Nearest neighbors |
High accuracy and robustness in brain tumor detection |
[64] |
Breast cancer |
MRI |
Deep learning |
Cascade deep learning network significantly improves detection efficiency |
[69] |
Multi-omics integration strategies combine genomic, epigenomic, proteomic, and imaging data to provide a comprehensive perspective for early cancer detection (Figure 2). This approach enables a more thorough capture of the biological characteristics of tumors, thereby improving both the sensitivity and specificity of detection. However, the integration and interpretation of multi-omics data present significant challenges, particularly in clinical translation. Ensuring data standardization and reproducibility of the results during this process requires further investigation.
Application of early detection in different types of tumors
The requirements and technological applications for early detection vary across different tumor types. For instance, early screening for breast cancer is relatively well established, with mammography (breast X-ray) and MRI being widely utilized [74, 75]. For lung cancer, Jonas DE demonstrated that low-dose spiral CT (LDCT) has been proven effective for screening high-risk populations [76]. Early detection of colorectal cancer typically relies on the fecal occult blood test (FOBT) and colonoscopy. However, in recent years, stool-based DNA methylation testing has also shown promising potential.
Challenges in clinical translation
Despite technological advancements that offer greater possibilities for early cancer detection, several challenges remain in their clinical application. First, it is critical to improve the specificity and sensitivity of detection methods to reduce false positives and negatives. False negatives can delay diagnosis and result in missed opportunities for optimal care, whereas false positives can lead to unnecessary follow-up tests and treatments, increasing both financial and psychological burdens on patients. Second, concerns regarding accessibility and cost-effectiveness hinder the widespread use of early detection systems. The high cost of these tests makes them difficult to implement in low resource areas. Additionally, further improvements are required in the standardization and clinical validation of emerging technologies, such as multi-cancer detection, to ensure their broad applicability and effectiveness across diverse populations [77].
Ultimately, there are technological barriers to the clinical adoption of cutting-edge technologies, such as liquid biopsy. Accurately identifying the tumor origin and integrating multi-omics data to enhance diagnostic accuracy remains a challenge. Overcoming these barriers will require extensive clinical studies and continued technological advancements [78]. Additionally, the integration of complex multi-omics datasets necessitates advanced computational tools and standardized protocols to ensure consistency and reliability in clinical practice.
Clinical applications of early detection
In clinical practice, early cancer identification is crucial as it can significantly increase patient survival rates and reduce treatment costs. Currently, some of the most widely used early screening methods include Pap smears for cervical cancer, colonoscopy for colorectal cancer, fecal occult blood tests for breast cancer, and low-dose spiral CT for lung cancer. These screening techniques have significantly reduced the cancer mortality rates. However, they also have drawbacks, such as high false-positive rates and the invasiveness of certain procedures [77].
In recent years, liquid biopsy has gained increasing popularity as an early cancer detection technique. Liquid biopsy enables noninvasive detection and captures tumor heterogeneity and dynamic changes by identifying biomarkers in the blood, such as circulating tumor cells (CTCs) and circulating tumor DNA (ctDNA). Traditional tissue biopsy has not been entirely replaced by liquid biopsy but currently serves as a complementary tool. This is largely due to limitations in sensitivity and specificity, which are particularly problematic when ctDNA levels are extremely low, making detection more challenging in the early stages of cancer [2].
Despite their great potential in early cancer diagnosis, liquid biopsies still face technological challenges in clinical applications. First, pure and efficient extraction of tumor-derived exosomes from complex physiological fluids, such as blood, remains difficult. Additionally, exosome identification is complicated by their significant variability, which differs based on the tumor source in terms of size, composition, and biological function. Therefore, improvements in exosome separation, identification techniques, and exploration of their specific applications across different cancer types are required.
Tumor-educated platelet (TEP) detection is notable for its high sensitivity and specificity, particularly in the early stages of cancer when changes in TEP RNA transcriptomes provide critical information for early diagnosis. Furthermore, TEP detection is relatively simple and requires minimal sample collection, particularly when combined with liquid biopsy. However, clinical challenges with TEPs remain, particularly the need to exclude transcriptome anomalies unrelated to cancer and to standardize RNA sequencing protocols. Further research is needed to confirm the specificity of TEPs across various cancer types for broader clinical applications.
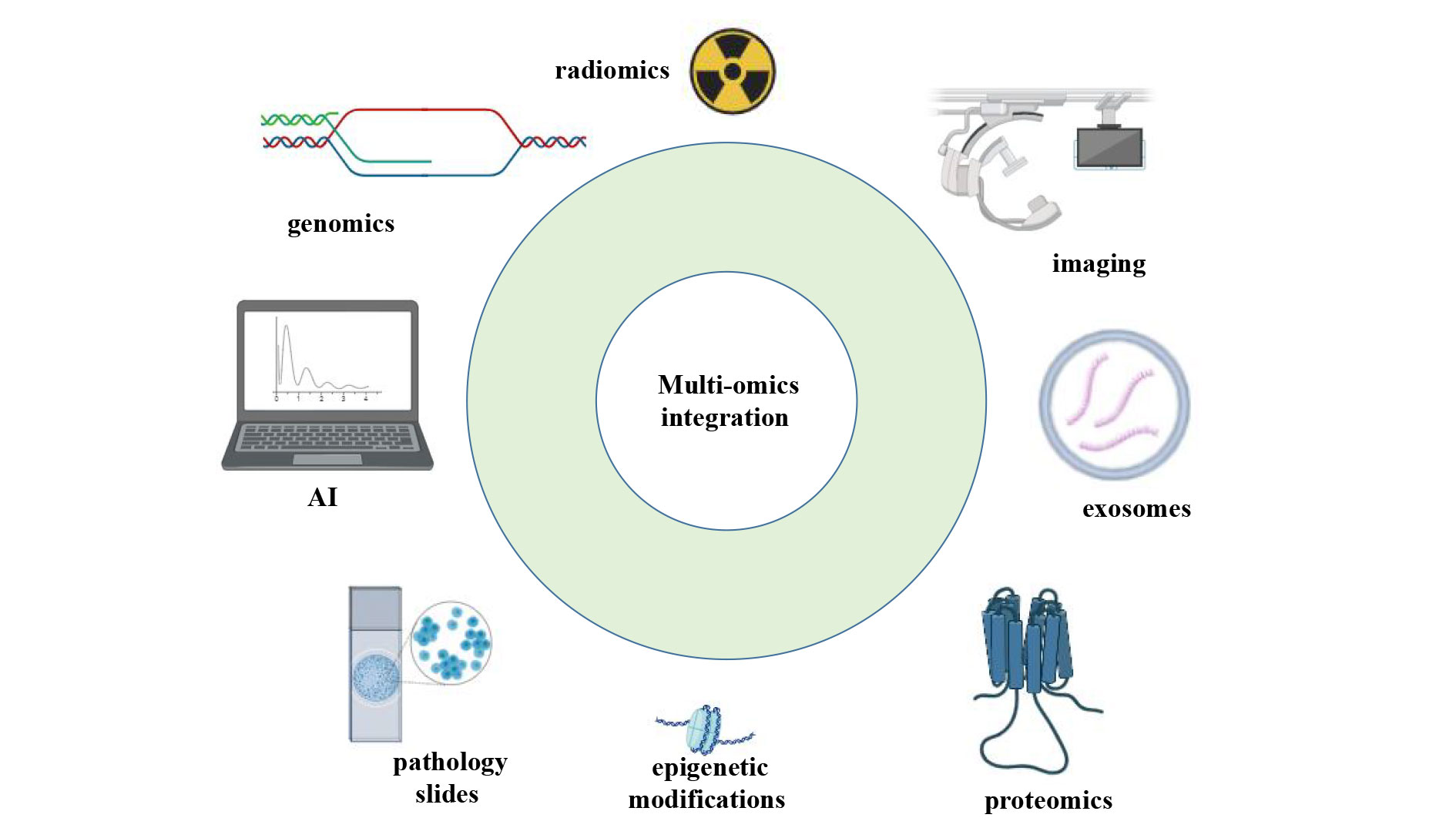
With advancements in precision medicine, personalized cancer detection strategies have become a major focus. By integrating patients' genomic information, epigenetic features, and lifestyle data, individualized screening and detection programs can be designed to identify early stage cancers more effectively while avoiding overdiagnosis and overtreatment. From a global health perspective, promoting and implementing early cancer detection technologies in low-income countries and regions is a critical research area. International collaboration and technology transfer are the key factors. By reducing costs, simplifying processes, and enhancing training, it will be possible to improve the early detection capabilities in these regions, ultimately reducing cancer-related mortality rates.
Exosomes and TEPs are two emerging biomarkers in liquid biopsies that offer new directions for early cancer detection. Future research should explore the combined application of these two markers, utilizing the molecular information within exosomes and the transcriptomic changes in TEPs to develop more sensitive, multidimensional early detection technologies. Furthermore, with the advancement of multi-omics analysis techniques, integrating exosomes and TEPs with ctDNA, CTCs, and other biomarkers is expected to improve the accuracy and comprehensiveness of early cancer diagnoses.
By studying the mechanisms of exosomes and TEPs and their specificity in various cancers, liquid biopsy has become a crucial tool for clinical cancer screening, early diagnosis, and longitudinal monitoring. This progress holds great promise for the advancement of precision oncology research.
Additionally, the development of novel biomarkers and the integration of multi-omics data analysis will continue to drive advancements in early tumor detection technologies, offering more precise diagnoses and more effective treatment options for patients.
No applicable.
Availability of data and materials
Data and materials are available on request from the authors.
Ethical policy
There is no any ethical events involved.
Author contributions
Rodney Bradly conceptualized, designed, conducted research, wrote the manuscript, and contributed to the revision and figure production.
Competing interests
There is no conflict of interest regarding the publication of this document.
Funding
None.
- Walter FM, Thompson MJ, Wellwood I, Abel GA, Hamilton W, Johnson M, Lyratzopoulos G, Messenger MP, Neal RD, Rubin G et al: Evaluating diagnostic strategies for early detection of cancer: the CanTest framework. BMC Cancer 2019, 19(1): 586.
- Singhi AD, Koay EJ, Chari ST, Maitra A: Early Detection of Pancreatic Cancer: Opportunities and Challenges. Gastroenterology 2019, 156(7): 2024-2040.
- Foser S, Maiese K, Digumarthy SR, Puig-Butille JA, Rebhan C: Looking to the Future of Early Detection in Cancer: Liquid Biopsies, Imaging, and Artificial Intelligence. Clin Chem 2024, 70(1): 27-32.
- Connal S, Cameron JM, Sala A, Brennan PM, Palmer DS, Palmer JD, Perlow H, Baker MJ: Liquid biopsies: the future of cancer early detection. J Transl Med 2023, 21(1): 118.
- Reese KL, Pantel K, Smit DJ: Multibiomarker panels in liquid biopsy for early detection of pancreatic cancer - a comprehensive review. J Exp Clin Cancer Res 2024, 43(1): 250.
- Nikanjam M, Kato S, Kurzrock R: Liquid biopsy: current technology and clinical applications. J Hematol Oncol 2022, 15(1): 131.
- Hosny A, Parmar C, Coroller TP, Grossmann P, Zeleznik R, Kumar A, Bussink J, Gillies RJ, Mak RH, Aerts H: Deep learning for lung cancer prognostication: A retrospective multi-cohort radiomics study. PLoS Med 2018, 15(11): e1002711.
- Milner DA, Jr., Lennerz JK: Technology and Future of Multi-Cancer Early Detection. Life (Basel) 2024, 14(7): 833.
- Kwon HJ, Park UH, Goh CJ, Park D, Lim YG, Lee IK, Do WJ, Lee KJ, Kim H, Yun SY et al: Enhancing Lung Cancer Classification through Integration of Liquid Biopsy Multi-Omics Data with Machine Learning Techniques. Cancers (Basel) 2023, 15(18): 4556.
- He X, Liu X, Zuo F, Shi H, Jing J: Artificial intelligence-based multi-omics analysis fuels cancer precision medicine. Semin Cancer Biol 2023, 88: 187-200.
- Nan Y, Ser JD, Walsh S, Schönlieb C, Roberts M, Selby I, Howard K, Owen J, Neville J, Guiot J et al: Data harmonisation for information fusion in digital healthcare: A state-of-the-art systematic review, meta-analysis and future research directions. Inf Fusion 2022, 82: 99-122.
- Underwood JC: More than meets the eye: the changing face of histopathology. Histopathology 2017, 70(1): 4-9.
- Dogan NU, Dogan S, Favero G, Köhler C, Dursun P: The Basics of Sentinel Lymph Node Biopsy: Anatomical and Pathophysiological Considerations and Clinical Aspects. J Oncol 2019, 2019: 3415630.
- Merriel SWD, Pocock L, Gilbert E, Creavin S, Walter FM, Spencer A, Hamilton W: Systematic review and meta-analysis of the diagnostic accuracy of prostate-specific antigen (PSA) for the detection of prostate cancer in symptomatic patients. BMC Med 2022, 20(1): 54.
- Ilic D, Djulbegovic M, Jung JH, Hwang EC, Zhou Q, Cleves A, Agoritsas T, Dahm P: Prostate cancer screening with prostate-specific antigen (PSA) test: a systematic review and meta-analysis. Bmj 2018, 362: k3519.
- Stejskal P, Goodarzi H, Srovnal J, Hajdúch M, van 't Veer LJ, Magbanua MJM: Circulating tumor nucleic acids: biology, release mechanisms, and clinical relevance. Mol Cancer 2023, 22(1): 15.
- Itani MM, Nassar FJ, Tfayli AH, Talhouk RS, Chamandi GK, Itani ARS, Makoukji J, Boustany RN, Hou L, Zgheib NK, Nasr RR: A Signature of Four Circulating microRNAs as Potential Biomarkers for Diagnosing Early-Stage Breast Cancer. Int J Mol Sci 2021, 22(11): 6121.
- Yu D, Li Y, Wang M, Gu J, Xu W, Cai H, Fang X, Zhang X: Exosomes as a new frontier of cancer liquid biopsy. Mol Cancer 2022, 21(1): 56.
- Lu Y, Han G, Zhang Y, Zhang L, Li Z, Wang Q, Chen Z, Wang X, Wu J: M2 macrophage-secreted exosomes promote metastasis and increase vascular permeability in hepatocellular carcinoma. Cell Communication and Signaling 2023, 21(1): 299.
- Tan S, Xia L, Yi P, Han Y, Tang L, Pan Q, Tian Y, Rao S, Oyang L, Liang J et al: Exosomal miRNAs in tumor microenvironment. Journal of Experimental & Clinical Cancer Research 2020, 39(1): 67.
- Jiang C, Zhang N, Hu X, Wang H: Tumor-associated exosomes promote lung cancer metastasis through multiple mechanisms. Molecular Cancer 2021, 20(1): 117.
- Best MG, Wesseling P, Wurdinger T: Tumor-Educated Platelets as a Noninvasive Biomarker Source for Cancer Detection and Progression Monitoring. Cancer Research 2018, 78(13): 3407-3412.
- Xu L, Li X, Li X, Wang X, Ma Q, She D, Lu X, Zhang J, Yang Q, Lei S et al: RNA profiling of blood platelets noninvasively differentiates colorectal cancer from healthy donors and noncancerous intestinal diseases: a retrospective cohort study. Genome Medicine 2022, 14(1): 26.
- Calverley DC, Phang TL, Choudhury QG, Gao B, Oton AB, Weyant MJ, Geraci MW: Significant downregulation of platelet gene expression in metastatic lung cancer. Clin Transl Sci 2010, 3(5): 227-232.
- Wiyarta E, Nugraha DA, Ramadani MI, Gustya GF, Ammar MF, Edwar HD, Kheirizzad N, Mukhlisah MN, Burhan E, Syahruddin E: Clinical utility and diagnostic value of tumor-educated platelets in lung cancer: a systematic review and meta-analysis. Front Oncol 2023, 13: 1201713.
- Zalis M, Viana Veloso GG, Aguiar PN, Jr., Gimenes N, Reis MX, Matsas S, Ferreira CG: Next-generation sequencing impact on cancer care: applications, challenges, and future directions. Front Genet 2024, 15: 1420190.
- Canino F, Tornincasa A, Bettelli S, Manfredini S, Barbolini M, Moscetti L, Omarini C, Toss A, Tamburrano F, Antonelli G et al: Real-World Data and Clinical Implications of Next-Generation Sequencing (NGS)-Based Analysis in Metastatic Breast Cancer Patients. Int J Mol Sci 2024, 25(5): 2490.
- Chen M, Zhao H: Next-generation sequencing in liquid biopsy: cancer screening and early detection. Hum Genomics 2019, 13(1): 34.
- Ribeiro M, Knebel FH, Bettoni F, Saddi R, Sacardo KP, Canedo F, Alessi JVM, Shimada AK, Marin JFG, Camargo AA, Katz A: Impressive response to dabrafenib, trametinib, and osimertinib in a metastatic EGFR-mutant/BRAF V600E lung adenocarcinoma patient. NPJ Precis Oncol 2021, 5(1): 5.
- Davies H, Bignell GR, Cox C, Stephens P, Edkins S, Clegg S, Teague J, Woffendin H, Garnett MJ, Bottomley W et al: Mutations of the BRAF gene in human cancer. Nature 2002, 417(6892): 949-954.
- Andrieux G, Das T, Griffin M, Straehle J, Paine SML, Beck J, Boerries M, Heiland DH, Smith SJ, Rahman R, Chakraborty S: Spatially resolved transcriptomic profiles reveal unique defining molecular features of infiltrative 5ALA-metabolizing cells associated with glioblastoma recurrence. Genome Med 2023, 15(1): 48.
- Baylin SB, Jones PA: A decade of exploring the cancer epigenome — biological and translational implications. Nature Reviews Cancer 2011, 11(10): 726-734.
- Jones PA, Baylin SB: The fundamental role of epigenetic events in cancer. Nat Rev Genet 2002, 3(6): 415-428.
- Liu C, Tang H, Hu N, Li T: Methylomics and cancer: the current state of methylation profiling and marker development for clinical care. Cancer Cell International 2023, 23(1): 242.
- Hu S, Tao J, Peng M, Ye Z, Chen Z, Chen H, Yu H, Wang B, Fan JB, Ni B: Accurate detection of early-stage lung cancer using a panel of circulating cell-free DNA methylation biomarkers. Biomark Res 2023, 11(1): 45.
- Shen Y, Wang D, Yuan T, Fang H, Zhu C, Qin J, Xu X, Zhang C, Liu J, Zhang Y et al: Novel DNA methylation biomarkers in stool and blood for early detection of colorectal cancer and precancerous lesions. Clin Epigenetics 2023, 15(1): 26.
- Oubaddou Y, Oukabli M, Fenniche S, Elktaibi A, Elochi MR, Al Bouzidi A, Qmichou Z, Dakka N, Diorio C, Richter A et al: BRCA1 Promoter Hypermethylation in Malignant Breast Tumors and in the Histologically Normal Adjacent Tissues to the Tumors: Exploring Its Potential as a Biomarker and Its Clinical Significance in a Translational Approach. Genes (Basel) 2023, 14(9): 1680.
- Wu Y, Tong Y, Zhang H, Li Y, Zhu X, Li M, Qiu L, Liu W, Mei S, Mao Y et al: A novel dual-target Septin9 methylation assay for improved detection of early-stage colorectal cancer and high-grade intraepithelial neoplasia. BMC Cancer 2024, 24(1): 916.
- Loomans-Kropp HA, Song Y, Gala M, Parikh AR, Van Seventer EE, Alvarez R, Hitchins MP, Shoemaker RH, Umar A: Methylated Septin9 (mSEPT9): A promising blood-based biomarker for the detection and screening of early-onset colorectal cancer. Cancer Res Commun 2022, 2(2): 90-98.
- Zhou M, Yu S, Liu Y, Shu S, Xu Y, Liu M, Ge Y, Fan H: H3K27me3 Inactivates SFRP1 to Promote Cell Proliferation via Wnt/β-Catenin Signaling Pathway in Esophageal Squamous Cell Carcinoma. Dig Dis Sci 2023, 68(6): 2463-2473.
- Monaghan L, Massett ME, Bunschoten RP, Hoose A, Pirvan PA, Liskamp RMJ, Jørgensen HG, Huang X: The Emerging Role of H3K9me3 as a Potential Therapeutic Target in Acute Myeloid Leukemia. Front Oncol 2019, 9: 705.
- Zhou M, Yu S, Liu Y, Shu S, Xu Y, Liu M, Ge Y, Fan H: H3K27me3 Inactivates SFRP1 to Promote Cell Proliferation via Wnt/β-Catenin Signaling Pathway in Esophageal Squamous Cell Carcinoma. Digestive Diseases and Sciences 2023, 68(6): 2463-2473.
- Ngollo M, Lebert A, Dagdemir A, Judes G, Karsli-Ceppioglu S, Daures M, Kemeny J-L, Penault-Llorca F, Boiteux J-P, Bignon Y-J et al: The association between Histone 3 Lysine 27 Trimethylation (H3K27me3) and prostate cancer: relationship with clinicopathological parameters. BMC Cancer 2014, 14(1): 994.
- Alix-Panabières C, Marchetti D, Lang JE: Liquid biopsy: from concept to clinical application. Sci Rep 2023, 13(1): 21685.
- Hu S, Tao J, Peng M, Ye Z, Chen Z, Chen H, Yu H, Wang B, Fan J-B, Ni B: Accurate detection of early-stage lung cancer using a panel of circulating cell-free DNA methylation biomarkers. Biomarker Research 2023, 11(1): 45.
- Wang Z, Xie K, Zhu G, Ma C, Cheng C, Li Y, Xiao X, Li C, Tang J, Wang H et al: Early detection and stratification of lung cancer aided by a cost-effective assay targeting circulating tumor DNA (ctDNA) methylation. Respiratory Research 2023, 24(1): 163.
- Liu W, Innocenti F, Chen P, Das S, Cook EH Jr, Ratain MJ: Interethnic difference in the allelic distribution of human epidermal growth factor receptor intron 1 polymorphism. Clin Cancer Res 2003, 9(3): 1009-1012.
- Correa-Aguila R, Alonso-Pupo N, Hernández-Rodríguez EW: Multi-omics data integration approaches for precision oncology. Mol Omics 2022, 18(6): 469-479.
- Li X, Du Y, Jiang W, Dong S, Li W, Tang H, Yi J, Zhou W, Zhang H: Integrated transcriptomics, proteomics and metabolomics-based analysis uncover TAM2-associated glycolysis and pyruvate metabolic remodeling in pancreatic cancer. Front Immunol 2023, 14: 1170223.
- Sun Z, Feng D, Jiang L, Tian J, Wang J, Zhu W: Integrated proteomic and metabolomic analysis of plasma reveals regulatory pathways and key elements in thyroid cancer. Mol Omics 2023, 19(10): 800-809.
- Shen SY, Singhania R, Fehringer G, Chakravarthy A, Roehrl MHA, Chadwick D, Zuzarte PC, Borgida A, Wang TT, Li T et al: Sensitive tumour detection and classification using plasma cell-free DNA methylomes. Nature 2018, 563(7732): 579-583.
- Lee G, Bang L, Kim SY, Kim D, Sohn K-A: Identifying subtype-specific associations between gene expression and DNA methylation profiles in breast cancer. BMC Medical Genomics 2017, 10(1): 28.
- Liu CJ, Zhang L, Sun Y, Geng L, Wang R, Shi KM, Wan JX: Application of CT and MRI images based on an artificial intelligence algorithm for predicting lymph node metastasis in breast cancer patients: a meta-analysis. BMC Cancer 2023, 23(1): 1134.
- Li H, Li L, Liu Y, Deng Y, Zhu Y, Huang L, Long T, Zeng L, Shu Y, Peng D: Predictive value of CT and 18F-FDG PET/CT features on spread through air space in lung adenocarcinoma. BMC Cancer 2024, 24(1): 434.
- Mallum A, Mkhize T, Akudugu JM, Ngwa W, Vorster M: The Role of Positron Emission Tomography and Computed Tomographic (PET/CT) Imaging for Radiation Therapy Planning: A Literature Review. Diagnostics (Basel) 2022, 13(1): 53.
- Gao F, Liu F, Wang J, Bi J, Zhai L, Li D: Molecular probes targeting HER2 PET/CT and their application in advanced breast cancer. J Cancer Res Clin Oncol 2024, 150(3): 118.
- Yeh R, O'Donoghue JA, Jayaprakasam VS, Mauguen A, Min R, Park S, Brockway JP, Bromberg JF, Zhi WI, Robson ME et al: First-in-Human Evaluation of Site-Specifically Labeled (89)Zr-Pertuzumab in Patients with HER2-Positive Breast Cancer. J Nucl Med 2024, 65(3): 386-393.
- Lo HZ, Choy KT, Kong JCH: FDG-PET/MRI in colorectal cancer care: an updated systematic review. Abdominal Radiology 2024, https://doi.org/10.1007/s00261-024-04460-z. Epub ahead of print.
- Lv L, Xin B, Hao Y, Yang Z, Xu J, Wang L, Wang X, Song S, Guo X: Radiomic analysis for predicting prognosis of colorectal cancer from preoperative 18F-FDG PET/CT. Journal of Translational Medicine 2022, 20(1): 66.
- Chen P, Scarpelli ML, Healey DR, Mehta S, Quarles CC: MRI and amino acid PET detection of whole-brain tumor burden. Front Oncol 2023, 13: 1248249.
- Anaya-Isaza A, Mera-Jiménez L, Verdugo-Alejo L, Sarasti L: Optimizing MRI-based brain tumor classification and detection using AI: A comparative analysis of neural networks, transfer learning, data augmentation, and the cross-transformer network. Eur J Radiol Open 2023, 10:100484.
- Archana KV, Komarasamy G: A novel deep learning-based brain tumor detection using the Bagging ensemble with K-nearest neighbor. J Intellig Sys 2023, 32(1).
- Vicini S, Bortolotto C, Rengo M, Ballerini D, Bellini D, Carbone I, Preda L, Laghi A, Coppola F, Faggioni L: A narrative review on current imaging applications of artificial intelligence and radiomics in oncology: focus on the three most common cancers. Radiol Med 2022, 127(8): 819-836.
- Scapicchio C, Gabelloni M, Barucci A, Cioni D, Saba L, Neri E: A deep look into radiomics. Radiol Med 2021, 126(10): 1296-1311.
- Alsheikhy AA, Said Y, Shawly T, Alzahrani AK, Lahza H: A CAD System for Lung Cancer Detection Using Hybrid Deep Learning Techniques. Diagnostics (Basel) 2023, 13(6): 1174.
- Feretzakis G, Juliebø-Jones P, Tsaturyan A, Sener TE, Verykios VS, Karapiperis D, Bellos T, Katsimperis S, Angelopoulos P, Varkarakis I et al: Emerging Trends in AI and Radiomics for Bladder, Kidney, and Prostate Cancer: A Critical Review. Cancers (Basel) 2024, 16(4): 810.
- Arooj S, Atta Ur R, Zubair M, Khan MF, Alissa K, Khan MA, Mosavi A: Breast Cancer Detection and Classification Empowered With Transfer Learning. Front Public Health 2022, 10: 924432.
- Nardone V, Reginelli A, Grassi R, Boldrini L, Vacca G, D'Ippolito E, Annunziata S, Farchione A, Belfiore MP, Desideri I, Cappabianca S: Delta radiomics: a systematic review. Radiol Med 2021, 126(12): 1571-1583.
- Zhang X, Zhang G, Qiu X, Yin J, Tan W, Yin X, Yang H, Wang H, Zhang Y: Exploring non-invasive precision treatment in non-small cell lung cancer patients through deep learning radiomics across imaging features and molecular phenotypes. Biomark Res 2024, 12(1): 12.
- García-Figueiras R, Baleato-González S, Padhani AR, Luna-Alcalá A, Vallejo-Casas JA, Sala E, Vilanova JC, Koh D-M, Herranz-Carnero M, Vargas HA: How clinical imaging can assess cancer biology. Insights into Imaging 2019, 10(1): 28.
- Hofman MS, Hicks RJ: How We Read Oncologic FDG PET/CT. Cancer Imaging 2016, 16(1): 35.
- Vijayakumar S, Yang J, Nittala MR, Velazquez AE, Huddleston BL, Rugnath NA, Adari N, Yajurvedi AK, Komanduri A, Yang CC et al: Changing Role of PET/CT in Cancer Care With a Focus on Radiotherapy. Cureus 2022, 14(12): e32840.
- Kim N, Cho H, Yun M, Park KR, Lee CG: Prognostic values of mid-radiotherapy 18F-FDG PET/CT in patients with esophageal cancer. Radiation Oncology 2019, 14(1): 27.
- Aymaz S: A new framework for early diagnosis of breast cancer using mammography images. Neural Computing and Applications 2024, 36(4): 1665-1680.
- Enogieru IE, Comstock CE, Grimm LJ: Breast Cancer Screening and Treatment Clinical Trials Updated for 2023. Journal of Breast Imaging 2023, 6(1): 14-22.
- Jonas DE, Reuland DS, Reddy SM, Nagle M, Clark SD, Weber RP, Enyioha C, Malo TL, Brenner AT, Armstrong C et al: Screening for Lung Cancer With Low-Dose Computed Tomography: Updated Evidence Report and Systematic Review for the US Preventive Services Task Force. JAMA 2021, 325(10): 971-987.
- Connal S, Cameron JM, Sala A, Brennan PM, Palmer DS, Palmer JD, Perlow H, Baker MJ: Liquid biopsies: the future of cancer early detection. Journal of Translational Medicine 2023, 21(1): 118.
- Zhu Z, Hu E, Shen H, Tan J, Zeng S: The functional and clinical roles of liquid biopsy in patient-derived models. Journal of Hematology & Oncology 2023, 16(1): 36.
Asia-Pacific Journal of Oncology
print ISSN: 2708-7980, online ISSN: 2708-7999
Copyright © Asia Pac J Oncol. This work is licensed under a Creative Commons Attribution-NonCommercial-No Derivatives 4.0 International (CC BY-NC-ND 4.0) License.