Review | Open Access
The role of miRNAs as biomarkers in cancer
Yifan Ou1, Zhen Ren2
1Basic Medical College, Air Force Military Medical University, Xi'an, Shaanxi, P.R. China.
2Ultrasonography Department, the First Affiliated Hospital, Air Force Military Medical University, Xi'an, Shaanxi, P.R. China.
Correspondence: Zhen Ren(Ultrasonography Department, the First Affiliated Hospital, Air Force Military Medical University, Changle West Road 169, Xi'an 710000, Shaanxi Province, P.R. China; Email: renzhen_1115@163.com).
Asia-Pacific Journal of Oncology 2024, 5: 66-75. https://doi.org/10.32948/ajo.2024.10.18
Received: 22 Sep 2024 | Accepted: 20 Oct 2024 | Published online: 22 Oct 2024
Key words microRNAs, cancer biomarkers, tumorigenesis, liquid biopsy, early cancer detection, personalized therapy
As a subclass of non-coding RNAs, microRNAs (miRNAs) have shown great promise as cancer research biomarkers in recent years. miRNA molecules regulate the translation and degradation of target genes, which is vital in various biological processes, including cell division, proliferation, apoptosis, and metabolism [3]. The miRNAs are closely associated with tumorigenesis and cancer progression. Numerous studies have demonstrated that dysregulation of miRNA expression is the key factor in cancer initiation, invasion, and metastasis [4, 5]. For example, unregulated cell proliferation promotes cancer development because oncogenes such as RAS are overexpressed, while tumor suppressor miRNAs such as let-7 are down-regulated [6]. miR-21 overexpression targets apoptosis and cytoskeleton regulation-related genes, including PDCD4 and TPM1, thereby increasing the motility and invasiveness of cancer cells during the invasive phase [7, 8]. Furthermore, it has been shown that miR-10b increases the invasiveness and motility of cancer cells during metastasis by blocking HOXD10 which involve in cell adhesion and extracellular matrix organization [9]. By controlling oncogenes or tumor suppressor genes, particular miRNAs can function as oncogenic drivers or as parts of tumor-suppressive systems. For instance, Javanmardi S. discovered that miR-21 is a well-known oncogenic miRNA that is overexpressed in many types of cancer [10]. On the other hand, miR-34a is a tumor-suppressor miRNA, typically downregulated in tumor cells [11].
The biological behavior of tumors can be reflected in the expression levels of miRNAs, with significant tissue and disease specificity. Compared to conventional tissue biopsies, miRNAs provide notable technological benefits. They can be detected in bodily fluids such as blood, urine, and saliva through liquid biopsy techniques due to their exceptional stability. This offers a viable method for the non-invasive screening of cancer [12]. Furthermore, miRNAs can express a tumor's molecular features, which makes them helpful for a variety of clinical tasks, including tumor subtype identification, tumor progression prediction, and therapy response evaluation. Consequently, miRNAs have the potential to offer direction for tailored therapeutic approaches.
Shen, J. et al. study on the early detection of lung cancer showed elevated plasma levels of miR-21 and miR-210. Conversely, patients with malignant solitary pulmonary nodules (SPNs) showed significantly lower levels of miR-486-5p than patients with benign SPNs and healthy controls. Research indicates that these miRNAs can effectively distinguish between healthy individuals and lung cancer patients, demonstrating high sensitivity and specificity [13]. In breast cancer, miRNAs such as miR-155 [14, 15] and miR-21 [16] are closely associated with tumor invasiveness and metastasis. Their expression can be used as a biomarker for early diagnosis and for monitoring therapeutic responses. Furthermore, by examining miRNA expression patterns, physicians can forecast tumor recurrence rates and survival outcomes because there is a substantial correlation between miRNA expression and patient prognosis.
MiRNAs hold considerable promise for cancer treatment and diagnosis, yet several challenges hinder their practical application. One major issue is the significant heterogeneity in miRNA expression, with variations not only between patients but also within different tumor sites. Moreover, additional refining of miRNA detection techniques is needed to guarantee reproducibility and consistency of results between laboratories. A critical area for future research involves the integration of miRNA biomarkers with other omics data, including proteomics, genomes, and epigenomics [17], to improve diagnostic sensitivity and accuracy. Consequently, miRNAs have significant promise for early cancer diagnosis, diagnostic subtyping, prognostic evaluation, and tailored therapy. In summary, The rapid progress in miRNA research as tumor biomarkers, coupled with advances in detection technologies and the integration of multi-omics data, suggests the growing importance of miRNAs in clinical oncology. This study focuses on the potential of miRNAs as tumor biomarkers and their applications in early diagnosis, prognostic assessments, and therapeutic interventions.
miRNA biogenesis and processing
The biogenesis and processing of miRNAs are complex and systematic, involving multiple stages and enzymes. The process begins with the transcription of primary miRNAs (primor-miRNAs) from the genome, typically facilitated by RNA polymerase II or III. First cleaved in the nucleus by the Drosha-DGCR8 complex, primor-miRNAs are lengthy RNA molecules with a distinctive hairpin structure. These precursor miRNAs, also known as pre-miRNAs, are roughly 70 nucleotides long [18]. These pre-miRNAs are transported to the cytoplasm by Exportin-5, where they are further processed by the enzyme Dicer into mature miRNA duplexes. One strand of the duplex (the guide strand) is loaded into the RNA-induced silencing complex (RISC), while the other strand is typically degraded [19]. Any dysregulation in this processing pathway can result in abnormal miRNA expression, subsequently affecting the regulatory functions of target genes. Such abnormalities play a significant role in the development of cancer and other diseases. For instance, impaired Drosha or Dicer function can cause widespread miRNA dysregulation and promote tumorigenesis.
Mechanism of gene regulation by miRNA
The primary function of miRNAs is to regulate gene expression by binding to target mRNAs, thereby inhibiting their translation or promoting their degradation. miRNA binding typically occurs at the 3' untranslated region (3' UTR) of the target mRNA, a process mediated by the RNA-induced silencing complex (RISC). The particular processes entail either mRNA degradation or translational suppression [19, 20]. Important biological processes connected to metabolic control require this gene-silencing mechanism. Protein translation is prevented when miRNA attaches partial complementarity to the 3' UTR of the target mRNA, preventing ribosome recognition and binding to the mRNA. Essential biological processes linked to the regulation of metabolism include this gene-silencing mechanism [21]. miRNAs can affect gene transcription indirectly in addition to directly affecting mRNA through their regulation of transcription factors or interactions with chromatin modification complexes. Moreover, miRNAs can control histone modifications and DNA methylation, which controls epigenetic gene expression [22].
The role of miRNA in cellular physiological processes
miRNAs are crucial regulators of gene expression, influencing various cellular physiological processes, including immune response, metabolism, apoptosis, cell division, and proliferation. miRNAs can precisely regulate cell proliferation and differentiation by altering genes associated with the cell cycle and differentiation. For instance, the miR-34 family, recognized as tumor suppressor RNAs, interacts with the tumor suppressor gene p53. These results are supported by Hermeking H.'s investigation into the functions of miR-34a and miR-34b/c in apoptotic responses in tumor and normal cells [23]. Additionally, Jauhari A. investigated the role of the miR-34 family in neuronal differentiation and identified p53 as a mediator of nerve growth factor (NGF)-induced miR-34a expression, which is involved in the differentiation of PC12 cells [24]. Recent studies have also demonstrated that miR-124 exerts a significant impact on neuronal differentiation. Gu X. and colleagues outlined the role and potential mechanisms of miR-124 in neurodevelopment [25]. In apoptosis regulation, miRNAs modulate the balance between cell survival and death by targeting apoptosis inhibitors or promoters, such as BCL2 or PTEN [26]. In metabolic processes, Tsai WC et al. demonstrated miR-122's regulatory influence on liver metabolism, underscoring miRNAs' essential role in metabolic stability [27]. In the immune system, miRNAs such as miR-146a play a critical role in modulating immune cell function and inflammatory responses [28]. Overall, as key regulators of gene expression, miRNAs participate in and maintain various cellular physiological processes through intricate gene regulatory networks. Dysregulation of these networks may lead to a range of diseases, including cancer, metabolic disorders, and immune-related diseases. As research progresses, understanding miRNA functions and mechanisms offers promising avenues for novel therapeutic strategies targeting these diseases.
The role of miRNAs in tumorigenesis is critical, as they can function either as oncogenes (oncomiRs) promoting tumor development or as tumor suppressor miRNAs inhibiting tumor progression. In tumors, overexpressed miRNAs may contribute to cancer progression by inhibiting tumor suppressor genes, while the downregulation of other miRNAs may suppress tumor formation by inhibiting oncogene expression. For example, miR-21 is considered an oncomiR, and its overexpression has been observed in various cancers, such as lung and breast cancer. miR-21 promotes cell proliferation and anti-apoptotic capacity by targeting tumor suppressor genes such as PTEN, PDCD4, and TPM1. By suppressing PTEN, a key negative regulator of the PI3K/AKT signaling pathway, miR-21 leads to increased cell survival and growth [29]. Inhibition of PDCD4, a pro-apoptotic protein involved in programmed cell death, further enhances cancer cell resistance to apoptosis [7]. Additionally, downregulation of TPM1, a cytoskeletal protein, contributes to enhanced cell motility and invasiveness, promoting tumor progression and metastasis[8].Peralta-Zaragoza, O. discovered that miR-21 post-transcriptionally downregulates PTEN expression to promote cell proliferation and cervical cancer cell survival [30], Qi, L. et al. found that the expression of miR-21 and its targets (PTEN, PDCD4, TM1) in breast ductal carcinoma in situ and invasive carcinoma is associated with squamous atypia [31], Xu LF et al. demonstrated that miR-21 simultaneously regulates multiple processes, such as enhanced cell proliferation, apoptosis, and tumor invasiveness, by targeting PTEN, RECK, and Bcl-2 in GSQCLC, suggesting a key role in the tumorigenesis and progression of lung squamous cell carcinoma [32]. Additionally, miR-155 is highly expressed as an oncogenic miRNA in various cancers, promoting tumor cell survival and immune evasion by targeting tumor suppressor genes such as SHIP1 and SOCS1 [33]. The miR-34 family, including miR-34a, miR-34b, and miR-34c, is one of the classical tumor-suppressor miRNAs, and its expression is downregulated in numerous cancers [34]. For instance, miR-34a is associated with the p53 pathway, and the absence of miR-34a can lead to tumorigenesis [35]. Moreover, the let-7 family of miRNAs is widely involved in tumor suppression by inhibiting the expression of oncogenes such as RAS and HMGA2. Research by Yan, L. et al. showed that depletion of H19 impairs, while its overexpression enhances the motility and invasiveness of tumor cells, a phenomenon mediated by let-7-regulated metastasis-promoting genes, including Hmga2, c-Myc, and Igf2bp3 [36]. The dysregulation of these miRNAs can provide insights into the molecular mechanisms underlying tumorigenesis.
The role of miRNAs in tumor invasion and metastasis
Tumor invasion and metastasis are the leading causes of mortality in cancer patients. miRNAs play a crucial role in tumor metastasis by regulating processes such as cell migration, invasion, and epithelial-mesenchymal transition (EMT). During EMT, epithelial cells transform, losing their polarity and adhesion to become mesenchymal cells with increased migratory and invasive abilities. Aberrant expression of miRNAs influences cytoskeletal remodeling, cell adhesion, and matrix degradation, making them critical regulators of tumor invasion and metastasis. For instance, miR-221 and miR-222 are widely recognized as key miRNAs promoting tumor proliferation and invasion. miR-221 and miR-222 facilitate rapid tumor cell proliferation by inhibiting the cell cycle inhibitors p27 and p57 [37]. Furthermore, Zhang CZ et al. found that miR-221 and miR-222 target PUMA, a pro-apoptotic factor, thereby inducing glioblastoma cell survival and further enhancing cancer cell resistance [38]. These findings suggest that miR-221/222 plays a pivotal role in cell cycle regulation, apoptosis, and survival pathways.
miR-10b promotes the invasion and metastasis of breast cancer cells by upregulating the RHOC gene. RHOC, a member of the Rho GTPase family, is involved in the dynamic regulation of the cytoskeleton and cell migration. Studies have shown that miR-10b promotes cancer cell migration and invasion by inhibiting the expression of the HOXD10 gene, which in turn activates RHOC. Subsequently, research by Du, J. demonstrated differential expression of miR-10b between hepatocellular carcinoma (HCC) and adjacent non-tumor tissues, with reduced miR-10b expression in HCC being associated with venous invasion. High RHOC expression levels were also linked to venous invasion in HCC, indicating that both miR-10b and RHOC are independent predictors of invasion and metastasis in HC [39]. These findings suggest that miR-10b and RHOC are metastasis factors in breast cancer and play critical regulatory roles in the invasion and metastasis of liver cancer.
Huang Q. discovered that miR-373 and miR-520c enhance the migratory capacity of tumor cells by regulating genes such as CD44 and EGFR. [40]. EGFR plays a key role in cell proliferation and survival signaling pathways. CD44, a cell adhesion molecule, is crucial for maintaining interactions between tumor cells and their microenvironment. In contrast to pro-migratory miRNAs, the miR-200 family (including miR-200a, miR-200b, and miR-200c) effectively inhibits tumor metastasis by directly suppressing ZEB1 and ZEB2, thereby blocking the epithelial-mesenchymal transition (EMT) process. ZEB1 and ZEB2 downregulate E-cadherin and promote EMT, enhancing the migratory and invasive capabilities of tumor cells. Through the maintenance of E-cadherin expression, the miR-200 family efficiently inhibits the spread of cancer by maintaining epithelial phenotype [41]. Additionally, miR-31 targets genes such as RHOA and WAVE3, which are involved in cytoskeletal remodeling and cell invasion, respectively. By downregulating these genes, miR-31 curbs the invasive and migratory characteristics of tumor cells, acting as a potential metastasis suppressor in various cancers. In conclusion, miRNAs are essential for the development of tumors and their spread (Figure 1).
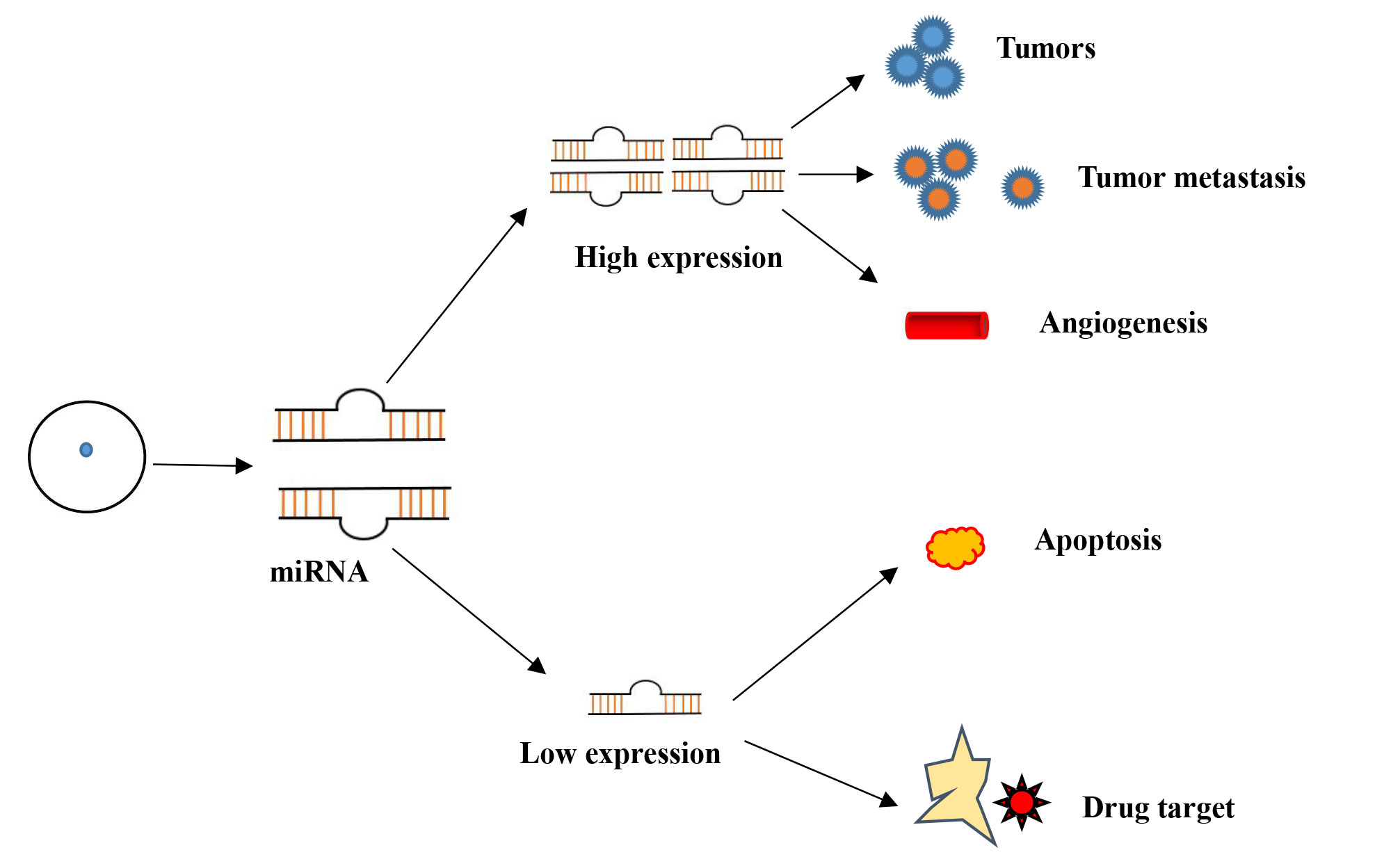
miRNA detection
Liquid biopsy, a non-invasive method, leverages biomarkers found in physiological fluids such as blood, urine, and saliva to facilitate early cancer diagnosis, monitor therapy, and evaluate recurrence. MiRNAs are a vital component of liquid biopsy because of their consistent presence in blood and other physiological fluids. The use of miRNAs has a number of benefits over traditional tissue biopsy, including non-invasiveness, high sensitivity, and great specificity. Of these, the early identification of cancer has shown considerable promise [43].
Compared to mRNA, miRNAs are highly stable and less prone to degradation by nucleases due to their ability to bind with proteins such as Argonaute or associate with microparticles. This association extends their half-life in external environments. Studies have demonstrated that miRNAs not only remain stable in plasma and serum but are also encapsulated and protected by exosomes, microvesicles, and other structures. This makes miRNAs reliable biomarkers for liquid biopsy, with potential applications in early screening and diagnosis of various cancer types. Currently, miRNAs, as biomarkers for liquid biopsy, have shown promising clinical applications in multiple cancer types. For instance, research by Porzycki P. et al. revealed an elevated level of the miR-141-3p, miR-21, and miR-375 relative expression in prostate cancer [44], closely correlating with the occurrence and progression of prostate cancer. miRNA detection not only aids in early cancer screening but also provides a basis for molecular subtyping and personalized treatment of tumors. Additionally, miRNAs are crucial in monitoring cancer recurrence and evaluating treatment efficacy. In breast cancer treatment, Müller V. et al. have found that variations in the levels of miR-21, miR-210, and miR-373 can indicate patient responses to chemotherapy and targeted therapy, showcasing the broad potential of miRNAs in cancer management [45].
Continuous technological advancements have led to breakthroughs in miRNA detection to enhance the sensitivity and specificity of miRNA detection in liquid biopsy. Commonly used methods for miRNA analysis include qPCR, digital PCR (dPCR), and next-generation sequencing (NGS). These techniques make it possible to find incredibly low levels of miRNAs in bodily fluids, which helps with early cancer detection and diagnosis. NGS is appropriate for large-scale screening since it can produce high-throughput data and simultaneously detect many miRNAs [46, 47], while qPCR is favored for clinical testing due to its low cost and simplicity. Moreover, digital PCR offers notable advantages in terms of sensitivity and quantitative precision, particularly for detecting very low amounts of miRNAs in the blood of cancer patients. For instance, detecting miR-21 levels in the serum of colorectal cancer patients via digital PCR has been shown to enable early detection with a sensitivity exceeding 85% [48].
Application of miRNAs in early cancer detection
miRNAs play a critical role in the early detection of cancer, enhancing therapy success rates and survival outcomes due to their unique expression patterns. However, the practical application of miRNAs in clinical settings faces several challenges, including variability in miRNA expression profiles among patients, lack of standardized detection methods, and difficulties in distinguishing between normal and cancer-related miRNA changes. Additionally, the stability of miRNAs in biofluids and the reproducibility of results across different platforms remain significant hurdles to their widespread clinical use [49, 50].These patterns enable the identification of subtle pathogenic changes at the initial stages of carcinogenesis, making miRNAs excellent biomarkers for early cancer diagnosis. Through liquid biopsy, miRNAs in body fluids allow for the early identification of tumor-related gene expression changes, opening avenues for timely intervention. Research has demonstrated that miRNAs not only sensitively capture molecular changes in tumors but also distinguish between different cancer types, providing valuable insights for personalized medicine.
Breast cancer is one of the most extensively studied fields in miRNA research. Elevated levels of miR-155, miR-21, and miR-10b in the blood of patients correlate strongly with breast cancer stage, invasiveness, and prognosis [51]. Zhang J. demonstrated that miR-10b expression is associated with disease stage, survival status, and tumor size [52]. Notably, miR-21, commonly overexpressed in various cancers, has emerged as one of the widely studied oncogenic miRNAs. Furthermore, Shang C. reported that the low expression of miR-127 contributes to breast cancer migration, invasion, and tumorigenesis, making it a potential therapeutic target and prognostic biomarker for breast cancer [53]. In early screening for breast cancer, Wang F. and colleagues found that miR-155 holds potential diagnostic value for breast cancer [15]. Additionally, previous research explored serum exosomal microRNA-21 (miR-21) as a biomarker for the early detection and diagnosis of breast cancer[54]. Both miR-155 and miR-21 could serve as potential diagnostic markers, aiding in the differentiation between precancerous lesions and malignant tumors and providing clinicians with more comprehensive diagnostic information.
Lung cancer, with high incidence and mortality, has shown promise for early detection through miRNA profiling. Studies have indicated that elevated levels of miRNAs such as miR-21, miR-486, and miR-210 in plasma can aid in identifying early-stage lung cancer patients [55]. Research by Li W. suggests that miR-486 and miR-150 may serve as potential blood-based biomarkers for the early diagnosis of non-small cell lung cancer (NSCLC). Monitoring changes in miR-486 expression could provide a non-invasive method for predicting recurrence in early-stage NSCLC [56]. Compared to traditional chest X-rays and CT scans, miRNA detection can diagnose tumors at earlier stages, even in cases of low tumor burden, as aberrant miRNA expression can still be sensitively detected. Furthermore, in lung cancer detection, the overexpression of miRNAs such as miR-17-5p and miR-19a has been closely associated with early tumor progression. Studies have shown that these miRNAs can help differentiate early-stage lung cancer patients from healthy controls. Yamamoto K. et al. identified FOXP1, TP53INP1, TNFAIP3, and TUSC2 as targets of miR-19a involved in lung cancer progression [57]. miRNA detection not only facilitates early cancer diagnosis but also assists in distinguishing between benign and malignant lesions, providing additional information for cancer screening.
In prostate cancer, miR-141 and miR-21 are two widely studied miRNAs. Previous research revealed that miR-141 is significantly elevated in the plasma of prostate cancer patients [58], and its expression level has been closely associated with the pathological staging and invasiveness of the disease. Furthermore, miR-21, a well-known oncogenic miRNA, is upregulated in prostate cancer and has potential utility in early diagnosis and therapeutic monitoring. Elevated miR-21 expression serves as a biomarker for early detection of prostate cancer and as a potential marker for monitoring treatment response. Ribas J. demonstrated that increased miR-21 expression enhances the growth of CaP tumors in vivo. Additionally, quantitative reverse transcription-PCR analysis showed elevated miR-21 expression in CaP tissues compared to adjacent normal tissues, suggesting miR-21 as a contributing factor to the CaP pathogenesis [59]. Detection of these miRNAs through liquid biopsy technologies offers the potential for early, non-invasive diagnosis of prostate cancer.
Significant progress has been made in the detection of miRNAs in gastrointestinal tumors. Research indicates that miR-223, miR-17-5p, and miR-20a levels are substantially higher in gastric cancer patients, with these miRNAs' expression levels closely linked to tumor size, stage, and prognosis. Wang M. demonstrated that circulating miR-17-5p/20a levels may serve as promising non-invasive molecular biomarkers for monitoring pathological progression, prognosis prediction, and chemotherapy response in gastric cancer [60]. Li L. found that miRNA-223-3p promotes gastric cancer proliferation, invasion, and metastasis by regulating ECT2 via the Wnt/β-catenin signaling pathway [61]. Furthermore, in colorectal cancer, Liu GH. identified miR-92a and miR-21 as potential biomarkers for early diagnosis [62]. Notably, when combined with other biomarkers, such as CEA (carcinoembryonic antigen), these miRNAs significantly enhance diagnostic sensitivity and specificity, offering the potential for early cancer detection.
In summary, the specific expression of miRNAs has demonstrated significant potential for clinical applications across various cancers. The dysregulation of miRNAs in lung, breast, liver, colorectal, and prostate cancer has been extensively studied and validated. Detecting these miRNAs expression levels facilitates early cancer diagnosis, classification, and monitoring of therapeutic efficacy. The broad application prospects of miRNAs as biomarkers provide an important theoretical foundation and clinical tools for the future of personalized cancer therapy.
Multiple miRNAs have been identified as strong prognostic markers in cancer. Below are several representative miRNAs and their prognostic roles:
(1) miR-21 is a well-studied oncogenic miRNA that is upregulated in various tumor types. Studies indicate that high expression of miR-21 is strongly associated with tumor aggressiveness and poor prognosis. High levels of miR-21 in colorectal, breast, and gastric cancers correlate with tumor recurrence and shorter disease-free survival. Research by Wang W. et al. suggests that miR-21 could be a valuable biomarker for predicting cancer prognosis in clinical settings [65]. Additionally, Kang W.'s study shows that high miR-21 expression predicts recurrence-free survival in stage II colorectal cancer [66]. MiR-21 also serves as a marker for predicting chemotherapy and targeted therapy responses, with changes in expression indicating patient responses to treatment. A decrease in miR-21 levels after treatment suggests a favorable prognosis, whereas sustained high levels may signal potential drug resistance or a risk of tumor recurrence.
(2) miR-155 is abnormally overexpressed in various cancers, including lymphoma and lung cancer. Studies have shown that elevated miR-155 expression is closely associated with increased tumor aggressiveness and metastatic risk, particularly in patients with poor prognosis. Van Roosbroeck K et al. identified the miR-155/TP53 feedback loop, which contributes to chemotherapy resistance [67]. Therefore, miR-155 holds significant value in predicting tumor recurrence and treatment resistance [68].
(3) miR-34a as a tumor-suppressor miRNA: Low expression of miR-34a has been observed in various tumor types, including breast cancer, lung cancer, and prostate cancer. miR-34a plays a critical role in tumor suppression by inhibiting tumor cell proliferation and inducing apoptosis. Shi Y. demonstrated that miR-34a can inhibit the growth of non-small cell lung cancer (NSCLC) [69]. Furthermore, its low expression is typically associated with poor prognosis, higher recurrence rates, and shorter survival times. Wu X. found that downregulation of miR-34a leads to head and neck cancer by upregulating the MET oncogene and modulating tumor immune evasion [70].
In conclusion, the clinical application of miRNAs is not limited to early cancer detection but also extends to serving as prognostic biomarkers, aiding in the assessment of disease progression and therapeutic response. For example, dynamic changes in the levels of miRNAs, such as miR-21 and miR-155 can be used to monitor patient response to treatment. Additional discoveries and applications are detailed in Table 1, including findings related to Liver Cancer [71-73], Pancreatic Cancer [74, 75], Bladder Cancer [76-78], Ovarian Cancer [79-81], etc. By regularly detecting the expression of specific miRNAs during treatment, clinicians can evaluate therapeutic efficacy in real time and adjust treatment regimens accordingly. With advancements in detection technologies, the potential of miRNAs in tumor prognosis is vast, particularly in personalized therapy and monitoring tumor recurrence, where miRNAs will become crucial tools (Table 1).
Table 1. Application of miRNAs in tumor diagnosis and prognosis. |
|||
Tumor type |
miRNA name |
Main function |
References |
Breast cancer |
miR-21, miR-210, miR-155, miR-10b |
Associated with recurrence and shorter disease-free survival; related to hypoxia conditions and invasiveness; related to treatment resistance and metastasis. |
[10-12], [34], [40], [44] |
Colorectal cancer |
miR-21, miR-31, miR-92a |
Associated with recurrence and shorter disease-free survival; predicts metastasis and recurrence; early diagnosis. |
[4], [43], [55], [59] |
Lung cancer |
miR-150, miR-210, miR-486, miR-17-5p, miR-19a |
Related to tumor invasiveness, metastasis, and treatment resistance; associated with hypoxic conditions and early diagnosis. |
[60-62] |
Prostate cancer |
miR-141, miR-221, miR-21, miR-34a |
Associated with pathological staging, invasiveness, and metastasis. |
[39, 40], [51, 52] |
Gastric cancer |
miR-21, miR-223, miR-17-5p, miR-20a |
Related to recurrence and used for prognosis evaluation; associated with tumor size and staging. |
[53, 54], [72] |
Lymphoma |
miR-155, miR-34a |
Associated with invasiveness, metastasis, and treatment resistance; promotes apoptosis and inhibits proliferation. |
[42], [60, 61] |
Liver cancer |
miR-221, miR-122, miR-224, miR-10b |
Related to tumor metastasis, invasiveness, early diagnosis, and recurrence. |
[15],[64-66] |
Pancreatic cancer |
miR-375, miR-21, miR-196a |
Related to proliferation, poor prognosis, recurrence, and metastasis risk. |
[67, 68] |
Bladder cancer |
miR-145, miR-9, miR-100, miR-182-5p/p27 |
Tumor suppressor miRNAs, regulate proliferation, invasiveness, and treatment resistance. |
[69-71] |
Ovarian cancer |
miR-200 family, miR-21, miR-199a |
Inhibits EMT (epithelial-mesenchymal transition), reducing metastasis risk; associated with progression, recurrence, and poor prognosis. |
[72-74] |
Firstly, expression exhibits significant heterogeneity across different patients and tumor types [82]. This heterogeneity not only exists between various cancer types but also within different subtypes of the same cancer and among individual patients. For instance, there are recognized molecular subtypes of breast cancer, including triple-negative, hormone receptor-positive and HER2-positive, every one of which has unique clinical and genetic characteristics [83]. Similarly, in lung cancer, heterogeneity is observed between non-small cell lung cancer (NSCLC) and small cell lung cancer (SCLC), as well as among subtypes like adenocarcinoma and squamous cell carcinoma [84]. Moreover, intra-tumor heterogeneity within individual patients can lead to different clonal populations with variable drug responses, as observed in renal cell carcinoma [85]. Such variability in expression could lead to considerable differences in diagnostic and prognostic outcomes across patients. Therefore, standardizing miRNA detection and analysis methods to ensure consistency and reproducibility across different laboratories is a key challenge that needs to be addressed in future research.
Secondly, although liquid biopsy technologies are convenient and promising for miRNA clinical applications, the sensitivity and specificity of miRNA detection must be enhanced. The detection of miRNAs in liquid biopsy is limited by their low abundance and interference from the complex background in body fluids. Thus, more sensitive detection techniques are needed to accurately capture dynamic changes in miRNA levels [86]. Additionally, integrating multi-omics data (such as genomics, transcriptomics, and proteomics) to enhance the diagnostic accuracy and predictive power of miRNA detection will be a critical direction for future research.
Lastly, while numerous studies have demonstrated the potential of miRNAs in early cancer detection, prognosis evaluation, and therapeutic monitoring, their application in clinical practice requires further clinical validation and large-scale clinical trials. In the future, the continued development of high-throughput sequencing technologies and bioinformatics is likely to unveil more miRNA regulatory mechanisms, facilitating their broader clinical application.
In conclusion, the expanding technological landscape and deepening understanding of miRNA functions suggest a promising future for miRNAs in personalized cancer therapy. The enhancement of detection technologies, standardization of clinical workflows, and integration of multi-dimensional omics data are pivotal. These developments will not only strengthen the role of miRNAs in early cancer diagnosis and prognosis evaluation but also position them as significant therapeutic targets in future cancer treatments.
None.
Availability of data and materials
Data and materials are available on request from the authors.
Ethical policy
Not applicable.
Author contributions
YFO, conceptualized, designed, conducted research, and wrote the manuscript; ZR contributed to the revision and figure production and approved the final submission.
Competing interests
None.
Funding
None.
- Global cancer burden growing, amidst mounting need for services. Saudi Med J 2024, 45(3): 326-327.
- Siegel RL, Giaquinto AN, Jemal A: Cancer statistics, 2024. CA Cancer J Clin 2024, 74(1): 12-49.
- Nalbant E, Akkaya-Ulum YZ: Exploring regulatory mechanisms on miRNAs and their implications in inflammation-related diseases. Clin Exp Med 2024, 24(1): 142.
- Ellakwa DE-S, Mushtaq N, Khan S, Jabbar A, Abdelmalek MA, Wadan A-HS, Ellakwa TE, Raza A: Molecular functions of microRNAs in colorectal cancer: recent roles in proliferation, angiogenesis, apoptosis, and chemoresistance. Naunyn Schmiedebergs Arch Pharmacol 2024, 397(8): 5617-5630.
- Annese T, Tamma R, De Giorgis M, Ribatti D: microRNAs Biogenesis, Functions and Role in Tumor Angiogenesis. Front Oncol 2020, 10: 581007.
- Johnson SM, Grosshans H, Shingara J, Byrom M, Jarvis R, Cheng A, Labourier E, Reinert KL, Brown D, Slack FJ: RAS is regulated by the let-7 microRNA family. Cell 2005, 120(5): 635-647.
- Asangani IA, Rasheed SA, Nikolova DA, Leupold JH, Colburn NH, Post S, Allgayer H: MicroRNA-21 (miR-21) post-transcriptionally downregulates tumor suppressor Pdcd4 and stimulates invasion, intravasation and metastasis in colorectal cancer. Oncogene 2008, 27(15): 2128-2136.
- Zhu S, Si ML, Wu H, Mo YY: MicroRNA-21 targets the tumor suppressor gene tropomyosin 1 (TPM1). J Biol Chem 2007, 282(19): 14328-14336.
- Ma L, Teruya-Feldstein J, Weinberg RA: Tumour invasion and metastasis initiated by microRNA-10b in breast cancer. Nature 2007, 449(7163): 682-688.
- Javanmardi S, Aghamaali MR, Abolmaali SS, Mohammadi S, Tamaddon AM: miR-21, An Oncogenic Target miRNA for Cancer Therapy: Molecular Mechanisms and Recent Advancements in Chemo and Radio-resistance. Curr Gene Ther 2017, 16(6): 375-389.
- Li WJ, Wang Y, Liu R, Kasinski AL, Shen H, Slack FJ, Tang DG: MicroRNA-34a: Potent Tumor Suppressor, Cancer Stem Cell Inhibitor, and Potential Anticancer Therapeutic. Front Cell Dev Biol 2021, 9: 640587.
- Preethi KA, Selvakumar SC, Ross K, Jayaraman S, Tusubira D, Sekar D: Liquid biopsy: Exosomal microRNAs as novel diagnostic and prognostic biomarkers in cancer. Mol Cancer 2022, 21(1): 54.
- Shen J, Liu Z, Todd NW, Zhang H, Liao J, Yu L, Guarnera MA, Li R, Cai L, Zhan M, Jiang F: Diagnosis of lung cancer in individuals with solitary pulmonary nodules by plasma microRNA biomarkers. BMC Cancer 2011, 11(1): 374.
- Liu X, Chang Q, Wang H, Qian H, Jiang Y: Discovery and function exploration of microRNA-155 as a molecular biomarker for early detection of breast cancer. Breast Cancer 2021, 28(4): 806-821.
- Wang F, Wang J, Zhang H, Fu B, Zhang Y, Jia Q, Wang Y: Diagnostic value of circulating miR-155 for breast cancer: a meta-analysis. Front Oncol 2024, 14: 1374674.
- Petrović N: miR-21 Might be Involved in Breast Cancer Promotion and Invasion Rather than in Initial Events of Breast Cancer Development. Mol Diagn Ther 2016, 20(2): 97-110.
- Almohaywi M, Sugita BM, Centa A, Fonseca AS, Antunes VC, Fadda P, Mannion CM, Abijo T, Goldberg SL, Campbell MC et al: Deregulated miRNA Expression in Triple-Negative Breast Cancer of Ancestral Genomic-Characterized Latina Patients. Int J Mol Sci 2023, 24(17): 13046.
- Yeom K-H, Lee Y, Han J, Suh MR, Kim VN: Characterization of DGCR8/Pasha, the essential cofactor for Drosha in primary miRNA processing. Nucleic Acids Res 2006, 34(16): 4622-4629.
- Davis BN, Hata A: Regulation of MicroRNA Biogenesis: A miRiad of mechanisms. Cell Communication and Signaling 2009, 7(1): 18.
- Davis-Dusenbery BN, Hata A: Mechanisms of control of microRNA biogenesis. J Biochem2010, 148(4): 381-392.
- Zhao C, Sun X, Li L: Biogenesis and function of extracellular miRNAs. ExRNA 2019, 1(1): 38.
- Szczepanek J, Tretyn A: MicroRNA-Mediated Regulation of Histone-Modifying Enzymes in Cancer: Mechanisms and Therapeutic Implications. Biomolecules 2023, 13(11): 1590.
- Hermeking H: The miR-34 family in cancer and apoptosis. Cell Death Differ 2010, 17(2): 193-199.
- Jauhari A, Singh T, Singh P, Parmar D, Yadav S: Regulation of miR-34 Family in Neuronal Development. Mol Neurobiol 2018, 55(2): 936-945.
- Gu X, Xu X, Jia C, Wang J, Zhang J, Gao Q, Chen J: Molecular Mechanisms Involved in the Regulation of Neurodevelopment by miR-124. Mol Neurobiol 2023, 60(7): 3569-3583.
- Abolfathi H, Arabi M, Sheikhpour M: A literature review of microRNA and gene signaling pathways involved in the apoptosis pathway of lung cancer. Respir Res 2023, 24(1): 55.
- Tsai WC, Hsu SD, Hsu CS, Lai TC, Chen SJ, Shen R, Huang Y, Chen HC, Lee CH, Tsai TF et al: MicroRNA-122 plays a critical role in liver homeostasis and hepatocarcinogenesis. J Clin Invest 2012, 122(8): 2884-2897.
- Zhu F, Yang T, Ning M, Liu Y, Xia W, Fu Y, Wen T, Zheng M, Xia R, Qian R et al: MiR-146a alleviates inflammatory bowel disease in mice through systematic regulation of multiple genetic networks. Front Immunol 2024, 15: 1366319.
- Meng F, Henson R, Wehbe-Janek H, Ghoshal K, Jacob ST, Patel T: MicroRNA-21 regulates expression of the PTEN tumor suppressor gene in human hepatocellular cancer. Gastroenterology 2007, 133(2): 647-658.
- Peralta-Zaragoza O, Deas J, Meneses-Acosta A, De la O-Gómez F, Fernández-Tilapa G, Gómez-Cerón C, Benítez-Boijseauneau O, Burguete-García A, Torres-Poveda K, Bermúdez-Morales VH et al: Relevance of miR-21 in regulation of tumor suppressor gene PTEN in human cervical cancer cells. BMC Cancer 2016, 16(1): 215.
- Qi L, Bart J, Tan LP, Platteel I, Sluis Tvd, Huitema S, Harms G, Fu L, Hollema H, Berg Avd: Expression of miR-21 and its targets (PTEN, PDCD4, TM1) in flat epithelial atypia of the breast in relation to ductal carcinoma in situ and invasive carcinoma. BMC Cancer 2009, 9(1): 163.
- Xu LF, Wu ZP, Chen Y, Zhu QS, Hamidi S, Navab R: MicroRNA-21 (miR-21) regulates cellular proliferation, invasion, migration, and apoptosis by targeting PTEN, RECK and Bcl-2 in lung squamous carcinoma, Gejiu City, China. PLoS One 2014, 9(8): e103698.
- Higgs G, Slack F: The multiple roles of microRNA-155 in oncogenesis. J Clin Bioinforma 2013, 3(1): 17.
- Fu J, Imani S, Wu M-Y, Wu R-C: MicroRNA-34 Family in Cancers: Role, Mechanism, and Therapeutic Potential. Cancers 2023, 15(19): 4723.
- Navarro F, Lieberman J: miR-34 and p53: New Insights into a Complex Functional Relationship. PLoS One 2015, 10(7): e0132767.
- Yan L, Zhou J, Gao Y, Ghazal S, Lu L, Bellone S, Yang Y, Liu N, Zhao X, Santin AD et al: Regulation of tumor cell migration and invasion by the H19/let-7 axis is antagonized by metformin-induced DNA methylation. Oncogene 2015, 34(23): 3076-3084.
- Amini S, Abak A, Sakhinia E, Abhari A: MicroRNA-221 and MicroRNA-222 in Common Human Cancers: Expression, Function, and Triggering of Tumor Progression as a Key Modulator. Lab Med 2019, 50(4): 333-347.
- Zhang CZ, Zhang JX, Zhang AL, Shi ZD, Han L, Jia ZF, Yang WD, Wang GX, Jiang T, You YP et al: MiR-221 and miR-222 target PUMA to induce cell survival in glioblastoma. Mol Cancer 2010, 9(1): 229.
- Du J, Wang Y, Fu H, Zheng X, Lin M, Guo M, Wei L: Clinical and pathological features of miR-10b and RHOC gene expression in hepatocellular carcinoma. Chin Sci Bulletin 2014, 59(19): 2249-2253.
- Huang Q, Gumireddy K, Schrier M, le Sage C, Nagel R, Nair S, Egan DA, Li A, Huang G, Klein-Szanto AJ et al: The microRNAs miR-373 and miR-520c promote tumour invasion and metastasis. Nat Cell Biol 2008, 10(2): 202-210.
- Korpal M, Kang Y: The emerging role of miR-200 family of microRNAs in epithelial-mesenchymal transition and cancer metastasis. RNA Biol 2008, 5(3): 115-119.
- Liu X, Papukashvili D, Wang Z, Liu Y, Chen X, Li J, Li Z, Hu L, Li Z, Rcheulishvili N et al: Potential utility of miRNAs for liquid biopsy in breast cancer. Front Oncol 2022, 12: 940314.
- Connal S, Cameron JM, Sala A, Brennan PM, Palmer DS, Palmer JD, Perlow H, Baker MJ: Liquid biopsies: the future of cancer early detection. J Transl Med 2023, 21(1): 118.
- Porzycki P, Ciszkowicz E, Semik M, Tyrka M: Combination of three miRNA (miR-141, miR-21, and miR-375) as potential diagnostic tool for prostate cancer recognition. Int Urol Nephrol 2018, 50(9): 1619-1626.
- Müller V, Gade S, Steinbach B, Loibl S, von Minckwitz G, Untch M, Schwedler K, Lübbe K, Schem C, Fasching PA et al: Changes in serum levels of miR-21, miR-210, and miR-373 in HER2-positive breast cancer patients undergoing neoadjuvant therapy: a translational research project within the Geparquinto trial. Breast Cancer Res Treat 2014, 147(1): 61-68.
- Cui W, Xuan T, Liao T, Wang Y: From sequencing to validation: NGS-based exploration of plasma miRNA in papillary thyroid carcinoma. Front Oncol 2024, 14: 1410110.
- Kluiver J, Niu F, Yuan Y, Kok K, van den Berg A, Dzikiewicz-Krawczyk A: NGS-Based High-Throughput Screen to Identify MicroRNAs Regulating Growth of B-Cell Lymphoma. In: Lymphoma: Methods and Protocols. https://doi.org/10.1007/978-1-4939-9151-8_12. Epub ahead of print., edn. Edited by Küppers R. New York, NY: Springer New York; 2019: 269-282.
- Basati G, Emami Razavi A, Abdi S, Mirzaei A: Elevated level of microRNA-21 in the serum of patients with colorectal cancer. Med Oncol 2014, 31(10): 205.
- Hayes J, Peruzzi PP, Lawler S: MicroRNAs in cancer: biomarkers, functions and therapy. Trends Mol Med 2014, 20(8): 460-469.
- Calin GA, Croce CM: MicroRNA signatures in human cancers. Nat Rev Cancer 2006, 6(11): 857-866.
- Baylie T, Kasaw M, Getinet M, Getie G, Jemal M, Nigatu A, Ahmed H, Bogale M: The role of miRNAs as biomarkers in breast cancer. Front Oncol 2024, 14: 1374821.
- Zhang J, Yang J, Zhang X, Xu J, Sun Y, Zhang P: MicroRNA-10b expression in breast cancer and its clinical association. PLoS One 2018, 13(2): e0192509.
- Shang C, Chen Q, Zu F, Ren W: Integrated analysis identified prognostic microRNAs in breast cancer. BMC Cancer 2022, 22(1): 1170.
- Li H, Tie X-j: Exploring research progress in studying serum exosomal miRNA-21 as a molecular diagnostic marker for breast cancer. Clin Transl Oncol 2024, 26(9): 2166-2171.
- Lobera ES, Varela MA, Jimenez RL, Moreno RB: miRNA as biomarker in lung cancer. Mol Biol Rep 2023, 50(11): 9521-9527.
- Li W, Wang Y, Zhang Q, Tang L, Liu X, Dai Y, Xiao L, Huang S, Chen L, Guo Z et al: MicroRNA-486 as a Biomarker for Early Diagnosis and Recurrence of Non-Small Cell Lung Cancer. PLoS One 2015, 10(8): e0134220.
- Yamamoto K, Ito S, Hanafusa H, Shimizu K, Ouchida M: Uncovering Direct Targets of MiR-19a Involved in Lung Cancer Progression. PLoS One 2015, 10(9): e0137887.
- Osipov ID, Zaporozhchenko IA, Bondar AA, Zaripov MM, Voytsitskiy VE, Vlassov VV, Laktionov PP, Morozkin ES: Cell-Free miRNA-141 and miRNA-205 as Prostate Cancer Biomarkers. Adv Exp Med Biol 2016, 924: 9-12.
- Ribas J, Ni X, Haffner M, Wentzel EA, Salmasi AH, Chowdhury WH, Kudrolli TA, Yegnasubramanian S, Luo J, Rodriguez R et al: miR-21: an androgen receptor-regulated microRNA that promotes hormone-dependent and hormone-independent prostate cancer growth. Cancer Res 2009, 69(18): 7165-7169.
- Wang M, Gu H, Wang S, Qian H, Zhu W, Zhang L, Zhao C, Tao Y, Xu W: Circulating miR-17-5p and miR-20a: molecular markers for gastric cancer. Mol Med Rep 2012, 5(6): 1514-1520.
- Li L, Liu P, He C, Xu C: miRNA-223-3p regulates ECT2 to promote proliferation, invasion, and metastasis of gastric cancer through the Wnt/β-catenin signaling pathway. J Cancer Res Clin Oncol 2023, 149(1): 121-134.
- Liu G-H, Zhou Z-G, Chen R, Wang M-J, zhou B, Li Y, Sun X-F: Serum miR-21 and miR-92a as biomarkers in the diagnosis and prognosis of colorectal cancer. Tumor Biology 2013, 34(4): 2175-2181.
- Tian B, Hou M, Zhou K, Qiu X, Du Y, Gu Y, Yin X, Wang J: A Novel TCGA-Validated, MiRNA-Based Signature for Prediction of Breast Cancer Prognosis and Survival. Front Cell Dev Biol 2021, 9: 717462.
- Braicu C, Buiga R, Cojocneanu R, Buse M, Raduly L, Pop LA, Chira S, Budisan L, Jurj A, Ciocan C et al: Connecting the dots between different networks: miRNAs associated with bladder cancer risk and progression. J Exp Clin Cancer Res 2019, 38(1): 433.
- Wang W, Li J, Zhu W, Gao C, Jiang R, Li W, Hu Q, Zhang B: MicroRNA-21 and the clinical outcomes of various carcinomas: a systematic review and meta-analysis. BMC Cancer 2014, 14(1): 819.
- Kang WK, Lee JK, Oh ST, Lee SH, Jung CK: Stromal expression of miR-21 in T3-4a colorectal cancer is an independent predictor of early tumor relapse. BMC Gastroenterology 2015, 15(1): 2.
- Van Roosbroeck K, Fanini F, Setoyama T, Ivan C, Rodriguez-Aguayo C, Fuentes-Mattei E, Xiao L, Vannini I, Redis RS, D'Abundo L et al: Combining Anti-Mir-155 with Chemotherapy for the Treatment of Lung Cancers. Clin Cancer Res 2017, 23(11): 2891-2904.
- Bayraktar R, Van Roosbroeck K: miR-155 in cancer drug resistance and as target for miRNA-based therapeutics. Cancer and Metastasis Reviews 2018, 37(1): 33-44.
- Shi Y, Liu C, Liu X, Tang DG, Wang J: The microRNA miR-34a inhibits non-small cell lung cancer (NSCLC) growth and the CD44hi stem-like NSCLC cells. PLoS One 2014, 9(3): e90022.
- Wu X, Cheng Y-SL, Matthen M, Yoon A, Schwartz GK, Bala S, Taylor AM, Momen-Heravi F: Down-regulation of the tumor suppressor miR-34a contributes to head and neck cancer by up-regulating the MET oncogene and modulating tumor immune evasion. J Exp Clin Cancer Res 2021, 40(1):70.
- Rong M, Chen G, Dang Y: Increased MiR-221 expression in hepatocellular carcinoma tissues and its role in enhancing cell growth and inhibiting apoptosis in vitro. BMC Cancer 2013, 13(1): 21.
- Li S, Yao J, Xie M, Liu Y, Zheng M: Exosomal miRNAs in hepatocellular carcinoma development and clinical responses. J Hematol Oncol 2018, 11(1): 54.
- Wen J, Friedman JR: miR-122 regulates hepatic lipid metabolism and tumor suppression. J Clin Invest 2012, 122(8): 2773-2776.
- Chen C, Demirkhanyan L, Gondi CS: The Multifaceted Role of miR-21 in Pancreatic Cancers. Cells 2024, 13(11): 948.
- Huang F, Tang J, Zhuang X, Zhuang Y, Cheng W, Chen W, Yao H, Zhang S: MiR-196a promotes pancreatic cancer progression by targeting nuclear factor kappa-B-inhibitor alpha. PLoS One 2014, 9(2): e87897.
- Fujii T, Shimada K, Tatsumi Y, Hatakeyama K, Obayashi C, Fujimoto K, Konishi N: microRNA-145 promotes differentiation in human urothelial carcinoma through down-regulation of syndecan-1. BMC Cancer 2015, 15(1): 818.
- Xu C, Zeng Q, Xu W, Jiao L, Chen Y, Zhang Z, Wu C, Jin T, Pan A, Wei R et al: miRNA-100 inhibits human bladder urothelial carcinogenesis by directly targeting mTOR. Mol Cancer Ther 2013, 12(2): 207-219.
- Xie F, Li Y, Wang M, Huang C, Tao D, Zheng F, Zhang H, Zeng F, Xiao X, Jiang G: Circular RNA BCRC-3 suppresses bladder cancer proliferation through miR-182-5p/p27 axis. Molecular Cancer 2018, 17(1): 144.
- Jo H, Shim K, Jeoung D: Potential of the miR-200 Family as a Target for Developing Anti-Cancer Therapeutics. International Journal of Molecular Sciences 2022, 23(11): 5881.
- Deng Y, Zhao F, Hui L, Li X, Zhang D, Lin W, Chen Z, Ning Y: Suppressing miR-199a-3p by promoter methylation contributes to tumor aggressiveness and cisplatin resistance of ovarian cancer through promoting DDR1 expression. J Ovarian Res 2017, 10(1): 50.
- Klicka K, Grzywa TM, Mielniczuk A, Klinke A, Włodarski PK: The role of miR-200 family in the regulation of hallmarks of cancer. Front Oncol 2022, 12: 965231.
- Petrovic N, Ergün S, Isenovic ER: Levels of MicroRNA Heterogeneity in Cancer Biology. Mol Diagn Ther 2017, 21(5): 511-523.
- Perou CM, Sørlie T, Eisen MB, van de Rijn M, Jeffrey SS, Rees CA, Pollack JR, Ross DT, Johnsen H, Akslen LA et al: Molecular portraits of human breast tumours. Nature 2000, 406(6797): 747-752.
- Herbst RS, Heymach JV, Lippman SM: Lung cancer. N Engl J Med 2008, 359(13): 1367-1380.
- Gerlinger M, Rowan AJ, Horswell S, Math M, Larkin J, Endesfelder D, Gronroos E, Martinez P, Matthews N, Stewart A et al: Intratumor heterogeneity and branched evolution revealed by multiregion sequencing. N Engl J Med 2012, 366(10): 883-892.
- Hou Z, Deng W, Li A, Zhang Y, Chang J, Guan X, Chang Y, Wang K, Wang X, Ruan J: A sensitive one-pot ROA assay for rapid miRNA detection. aBIOTECH 2024, 5(3): 298-308.
Asia-Pacific Journal of Oncology
print ISSN: 2708-7980, online ISSN: 2708-7999
Copyright © Asia Pac J Oncol. This work is licensed under a Creative Commons Attribution-NonCommercial-No Derivatives 4.0 International (CC BY-NC-ND 4.0) License.