Review | Open Access
Next frontier in cancer treatment: an iPSC supported CAR-T cell therapies
Tayyaba Sona1
1Applied health science college, Hamad Bin Khalifa University, Doha, 5825, Qatar.
Correspondence: Tayyaba Sona (Applied health science college, Hamad Bin Khalifa University, Doha, 5825, Qatar; Email: tayyabasona@yahoo.com).
Asia-Pacific Journal of Oncology 2024, 5: 104-111. https://doi.org/10.32948/ajo.2024.12.23
Received: 18 Nov 2024 | Accepted: 23 Dec 2024 | Published online: 27 Dec 2024
Key words induced pluripotent stem cell (iPSC), chimeric antigen receptor (CAR)-T (CAR-T) cells, cancer therapy, allogeneic cell therapy, genetic engineering, graft-versus-host disease (GvHD), T cell receptor (TCR)
Treatment often results in adverse effects like headaches, myalgia, and pyrexia, highlighting the importance of vigilant patient care [10]. In the case of autologous CAR-T cell therapy, CAR receptors are introduced through genetic modification of patients own T cells, but scalability is constrained due to the limited availability of T cells for redosing. Conversely , in allogeneic CAR-T therapy the requirement for T cell is met from healthy donors/ stems cells, offer the possibility of an unlimited supply of therapeutic cells (Figure 1) [11]. However, these cells have shown reduced longevity in the body, increased risk of donor cells attack on the recipient tissue a condition called graft-versus-host disease (GvHD). Cutting-edge technologies would be need to overcome the above shortcomings of allogeneic CAR-T cell therapies [12].
CAR-T technology widespread application faces challenges owing to the rarity of antigen-specific human T lymphocytes, prompting the need for novel approaches to increase T-cell supply and applicability [13]. To overcome this problem and enable sustainable supply of “off the shelf” CAR -T cells induced pluripotent stem cells (iPSCs) technology harnessing stem cells has caused considerable excitement. iPSCs technology can be utilized to produce an abundant supply of functional T cells, addressing current limitations [14]. Importantly, peripheral blood T lymphocytes from healthy donors can also undergo transduction to create clones in the form of induced pluripotent stem cell [15].
The unique capabilities of iPSC technology enable the creation of diverse immune cells, including T cells. A significant breakthrough is the iPSC-derived EZ-T cells generation, which replicate the differentiation process of naive T cells into effector- and memory-like T cell subsets [16]. Recent research has successfully produced iPSCs assisted allogeneic CAR-T cells, as demonstrated by 1928z-T-iPSC-T, CAR-iPSC T cells, and T-iPSCs15 [17]. These studies highlight the potential, safety, and therapeutic effectiveness of iPSC-derived CAR-T cells [17]. However, several obstacles persist. The inefficiency of procedure through which differentiated cells are transformed into iPSCs has constrained the progress. Furthermore, the presence of numerous iPSC lines lacking authentic pluripotency exacerbates this issue, as they show insufficient differentiation potential across crucial embryonic cell lineages. iPSC assisted T-cell therapies maximum effectiveness can only be realized once these shortcoming are addressed [18].
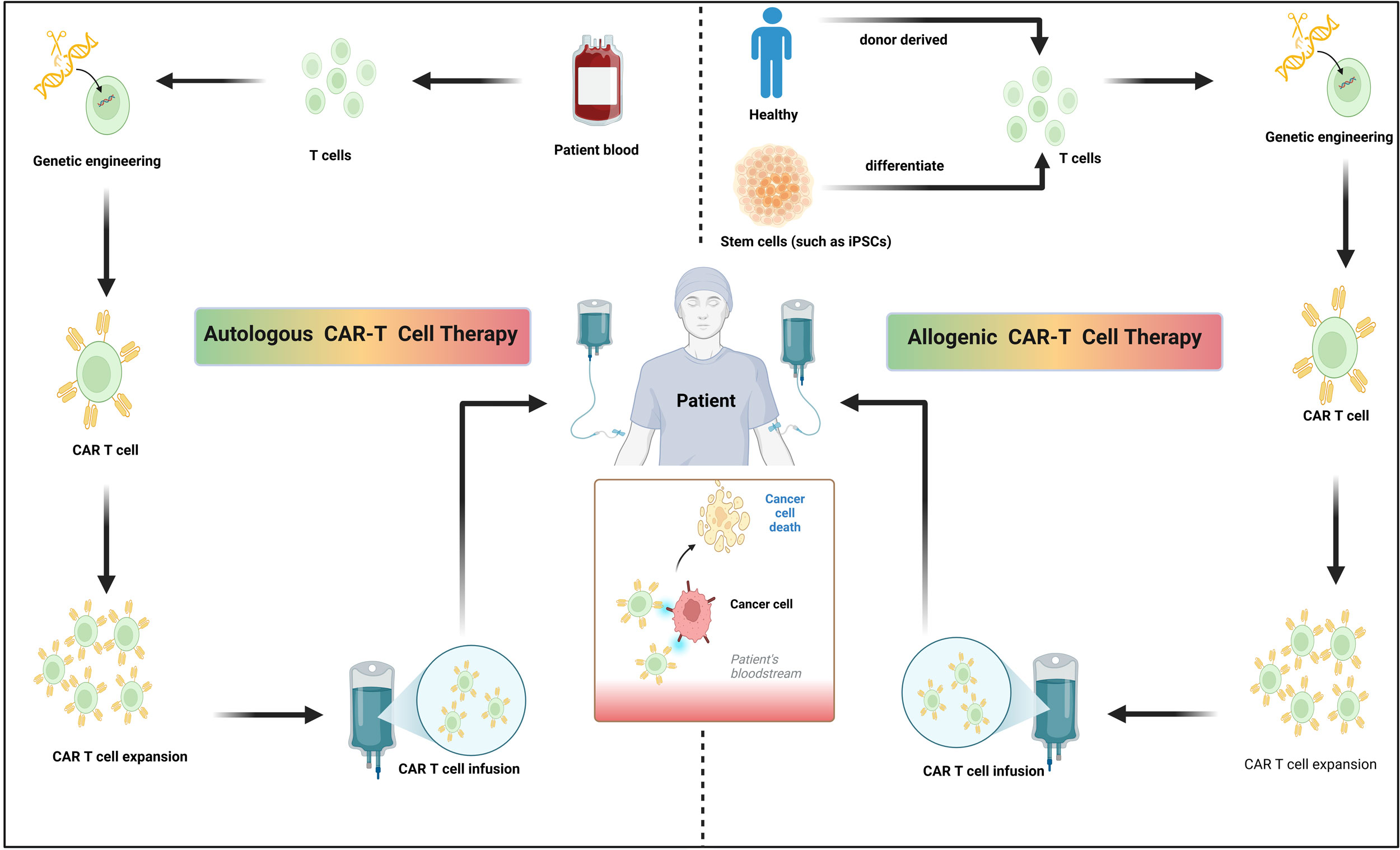
Autologous CAR-T cell therapy
CAR-T platform particularly against hematological malignancies has been hailed for its exceptional effectiveness. In particular, highly effective therapy against B-cell Acute Lymphoblastic Leukemia (B-ALL) [19] and multiple myeloma (MM), effectively treated 90% B-ALL patients and 80% MM patients, respectively [20]. Furthermore, CAR-T therapies for various other cancers are undergoing trails at preclinical and clinical stages, such as mesothelin-targeting CAR-T cells for ovarian cancer and CD70-targeting CAR-T cells for acute myeloid leukemia (AML) [21]. At present, multiple generations of CAR-T cell therapy are available [22]. The first generation of CARs consists of composite proteins with an external antigen-binding domain (typically an antibody's single-chain variable fragment) coupled to an internal signaling domain, often the CD3ζ chain from the T-cell receptor. Second-generation CARs enhance the efficacy of CAR-T cells by incorporating a costimulatory domain, such as CD28 or 4-1BB, along with CD3ζ. This fusion seeks to enhance the expansion and longevity of engineered cells within the body [23]. Third-generation CARs that incorporate multiple costimulatory domains have also been effectively devised [24]. Autologous second-generation CAR-T cells have achieved unprecedented success in achieving repeated complete remissions for patients with hematological malignancies previously deemed untreatable. This remarkable progress has resulted in the approval of tisagenlecleucel and axicabtagene ciloleucel, two groundbreaking therapies for treating relapsed or refractory B-ALL diffuse large B-cell lymphoma, and primary mediastinal large B-cell lymphoma. Thus, the creation of CAR-T cell platforms has transformed cancer treatment [25].
Currently, all available CAR-T therapies are autologous, meaning they use cells sourced from the patient. This inherent characteristic restricts the frequent use of this treatment can due to scarcity of such cells. As a result, comprehensive treatment of all cancer patients becomes a significant challenge. The manufacturing process itself presents substantial obstacles, including intricate logistical issues, prolonged intervals between leukapheresis to CAR-T cell infusion, heterogeneity in T-cell profiles influenced by individual immune system characteristics, and the effects of previous therapeutic interventions. Furthermore, the financial burden associated with the autologous method is exceptionally high, with potential costs reaching $373,000 per treatment course.
Allogenic CAR-T cell therapy
Allogeneic CAR-T cells could prove a key alternative for addressing the existing shortcoming. This approach allows for the treatment of multiple patients simultaneously [26]. These cells, derived from healthy donors, can provide an essentially unlimited supply. The generation process of allogeneic CAR-T cells is streamlined and efficient with opportunities for industrial scale-up. Through this method from a single donor a plenty of CAR-T cells can be generated that can be cryopreserved [27]. Furthermore, allogeneic CAR-T therapy is cost effective compared to its autologous counterpart. Despite its advantages, allogeneic CAR-T therapy poses substantial risks and obstacles [28]. The primary concern is graft-GvHD which results from the recipient's body rejecting donor cells from an unrelated, healthy individual. This triggers a series of immune responses in recipients [29]. GvHD is caused by differences in the MHC between the donor and recipient cells. Various approaches, including T cells receptors (TCR) disruption, were proposed to reduce the occurrence and severity of GvHD. Additionally, the utility of allogeneic CAR-T platform tends to be limited to short or intermediate periods [30]. In comparison, autologous treatments typically demonstrate longer-lasting effects, which persist for months to weeks [31].
Allogeneic TCR-disrupted CAR-T cell therapy. Allogeneic TCR-disrupted CAR-T cell therapy showed invaluable performance compared to traditional methods. TCRs and CARs are essential entities for improving immune cell targeting in stem cell engineering research. By employing immune cells extracted from PBMCs or stem cells in conjunction with genome editing vectors, TCRs and CARs can be permanently integrated into stem cells, thereby offering a sustained therapeutic solution [32]. This approach provide potent remedy for mitigating a key weakness of conventional allogeneic CAR-T therapy, its short-lived effectiveness [11].
CAR-T cells with disrupted TCRs can reduce or mitigate GvHD a side effect linked to allogeneic CAR-T therapy [33]. GvHD could be mitigated through TCR modification by genetic editing or using immune cells with specific sensitivities or non-responsiveness to peptide-MHC differences. Eliminating TCR gene function prevents T cell-induced GvHD. For example, knocking out the TCR gene eliminates host immune recognition and prevents subsequent reactions, particularly GvHD onset [34].
To exemplify, eliminating specific genes like B2M and CIITA through targeted knockout can prevent host T cell-mediated allo-rejection. Moreover, selective inactivation of immune checkpoint genes (such as PDCD1, LAG3, CTLA4, and DGKa) enhances tumoricidal ability of immune cells, boosting their capacity to suppress tumors [35]. This genetic manipulation improves the immune cells' capability to combat tumors [36]. CRISPR genome editing enables CAR genes introduction into the TCR locus, resulting in consistent CAR expression among T cells while simultaneously suppressing endogenous TCR expression, thus enhancing T cell efficacy [37]. Furthermore, genome-wide screening of therapeutic immune cells using CRISPR-Cas9 presents an effective approach for identifying gene targets relevant to cell-based therapeutic intervention [38].
Limitations of current CAR-T cell therapy
Complex genetic modifications offer promising potential for advanced modifications; however, they have potential drawbacks [39]. These include a higher frequency of aneuploidy, increased risk of tumor formation, reduced effectiveness against tumors in vivo, and decreased longevity of therapeutic cells. Recent studies have highlighted the significance of preserving TCR expression in CAR-T cell therapies. Production of cytokines is curtailed and diminished long-term persistence was observed in TCR-knockout CAR19-T cells distinct from CAR19-T cells with intact TCRs [40]. Therefore, novel approaches are essential for enhancing allogeneic CAR-T cell development. In particular, methods utilizing stem cell-derived techniques, such as iPSCs, would prove revolutionary in curtailing the weaknesses of allogenic CAR-T cell therapy for enhancing its effectiveness [41].
The need for undergoing prior lymphodepletion for CAR-T cell intervention introduces an additional limitation due to its associated toxicity. To mitigate this issue, it is crucial to enhance allogeneic CAR-T cells by restricting lymphodepletion and consolidation cycles. Furthermore, there are constraints on the practicality of administering multiple doses owing to prior need for repeated lymphodepleting to CAR-T cell infusion, primarily because of associated toxicities. This issue stems from the requirement for moderate-intensity conditioning protocols to promote CAR-T cell homeostatic expansion. In order to mitigate this, it may be necessary to develop genetically modified advanced allogeneic cells capable of evading the immune system of host [42]. Finally, the exhaustible reservoir of these cells presents a potential impediment, demanding comprehensive strategies for ex vivo expansion [43].
Table 1. List of CAR-T cell interventions with FDA approval [5]. |
|||
Generic name |
Brand name |
Target antigen |
Target disease |
Tisagenlecleucel |
Kymriah
|
CD19 |
B-cell precursor acute lymphoblastic leukemia (ALL) |
Axicabtagene ciloleucel |
Yescarta
|
CD19 |
Large B-cell lymphoma |
Brexucabtagene autoleucel |
Tecartus
|
CD19 |
Mantle cell lymphoma (MCL |
Lisocabtagene maraleucel |
Breyanzi
|
CD19 |
Large B-cell lymphoma |
Idecabtagene vicleucel |
Abecma
|
BCMA |
Multiple myeloma |
Ciltacabtagene autoleucel |
Carvykti
|
BCMA |
Multiple myeloma |
Obecabtagene autoleucel |
Aucatzyl |
CD19 |
B-cell acute lymphoblastic leukemia (ALL) |
Generate iPSC-derived CAR-T cells using an OP9-DL1 feeder-dependent culture
Previous research has successfully developed iPSC-derived CAR-T cells against CD19 as a cure for B-cell malignancies and infectious diseases. This study underscores a key weakness of T cell therapies: the limited quantity of T cells. Traditional adoptive T cell therapies depend on sophisticated operation involving donor selection and laborious generation of T cell lines or genetic manipulation of autologous T cells from individual patients, limiting the widespread application of therapies with predetermined antigen specificity. In contrast, iPSC-derived CAR-T cells, with excellent tumoricidal capabilities, are a promising alternative. Although pluripotent stem cells can serve as inexhaustible supply of T cells, however for absolute benefit from therapeutic potential of these iPSC-derived lymphoid cells additional research would be needed in this direction [44].
The researchers employed a novel approach by integrating iPSC and CAR engineering technology to generate human T cells with specific targeting capabilities for CD19. An antigen with prevalent expression in B cell malignancies. The engineered T cells, derived from iPSCs and equipped with CARs, exhibited characteristics akin to those of innate γδ T cells. When compared to CAR-transduced γδ T cells obtained from PBMNC, the iPSC-derived T cells showed strong tumoricidal effects in a xenograft model [45]. These findings indicate that second-generation CARs obtained through genetically manipulated iPSCs could offer an effective strategy to harness iPSCs inexhaustible supply potential, aiding manufacture of functional T cells with customized attributes and precise antigen-targeting abilities [44]. Scientists have converted healthy donor’s peripheral blood T lymphocytes into iPSCs through the introduction of retroviral vectors containing the transcription factors KLF4, SOX2, OCT4, and c-MYC13. This method is particularly important, as it presents the possibility of creating an endless source of T lymphocytes with specific antigen targeting, mitigating the requirement of HLA matching. By combining the iPSCs technology versatility with CAR technology, this approach could aid in manufacturing of various T-cell subtypes with required genetic modifications, thereby broadening its potential applications across different therapeutic fields [46].
Generate iPSC-derived CAR-T cells using a stroma-free culture
A recent investigation highlighted the potential of iPSCs as a versatile and rich reservoir for cellular therapies. This study shows that suppressing the EZH1 gene substantially improves the effective in vitro generation of iPSCs assisted T cells. Researchers have successfully created iPSC assisted T cells, dubbed EZ-T cells, by combining EZH1 knockdown-induced epigenetic alteration with a stroma-free T cell differentiation method. These EZ-T cells exhibit an extensively heterogeneous TCR repertoire and display molecular attributes akin to conventional αβ T cells extracted from peripheral blood. Upon stimulation, EZ-T cells evolve into both effector and memory T cell subsets. Additionally, introduction of CARs into EZ-T cells confers them with strong antitumor activity both in-vitro and ex-vivo. This research introduces a novel stroma-free method for generating T cells from iPSCs with pre-engineered TCRs or obtained from antigen-specific cytotoxic T cells. These specialized TCR-containing iPSCs showed distinct differentiation patterns compared to unmodified cells. To assess the potency of stroma-free technique, T cell differentiation was performed using both this innovative approach and a conventional OP9-DL1 co-culture system. Notably, increased CD3+ T-cell production was noticed for the stroma-free protocol [16].
Earlier investigations revealed that EZH1 plays a regulatory function in the preservation of hematopoietic multipotency [47]. Studies using mouse and zebrafish models have shown that EZH1 inhibition enhances lymphoid lineage potential during embryonic development. Expanding on these findings, it can be noted that EZH1 inhibition affects the in vitro differentiation of T cells from iPSCs [48]. This was accomplished by utilizing shRNA to suppress EZH1 expression during the differentiation of iPSC-derived CD34+ hematopoietic stem and progenitor cells into T cells. EZ-T cells exhibited improved effector characteristics, with notably higher CD69 expression in response to PMA/ionomycin stimulation than iPSC-OP9-T and iPSC-SF-T cells, when investigated through functional assays. Additionally, EZ-T cells showed increased CD107a expression relative to iPSC-SF-T controls under similar experimental conditions. These results contribute to the ongoing refinement of in vitro methods for generating T cells from iPSCs, guided by an enhanced understanding of the crucial signaling pathways involved in T cell development [49].
Generate iPSC-derived CAR-T cells using a 3D-organoid culture
A recent study examined the feasibility of creating an inexhaustible reservoir of CAR-T cells stemming from iPSCs to facilitate "off-the-shelf" CAR-T cell immunotherapy. This research tackles the obstacles in efficiently converting iPSCs into conventional αβ T cell lineages while preserving CARs expression and functionality. Scientists have successfully transformed iPSCs from CD62L+ naïve and memory T cells. Incorporated CD19-targeting CARs and employed 3D-organoid culture system for differentiation [50]. Yielded iPSC-derived CD8αβ-positive CAR-T cells that bear a strong resemblance to conventional CD8αβ-positive CAR-T cells. CD19-CAR-T cells stemming from iPSCs exhibited similar levels of antigen-specific activation, degranulation, cytotoxicity, and cytokine secretion as the traditional CAR-T cells. Moreover, these iPSC-derived cells consistently expressed TCR from the initial T cell clone. Further experiments in animal models ratified D19-CAR-T cells stemming from iPSCs potency for triggering tumoricidal responses. Mice implanted with CD19-positive human tumor xenografts exhibited good longevity. This research highlights the weakness of existing autologous CAR-T cell manufacturing processes, which rely on individualized blood apheresis and custom production steps. To address these issues, fully functional and developed human T-cells from hematopoietic stem cells (HSCs) and embryonic stem cells (ESCs) were generated by employing an 3D organoid culture system [51, 52]. This research further delved deeper into the functions of CD19-CAR-T cells derived from reprogrammed iPSCs, specifically examining their ability to target CD19-positive cells. The study also analyzed the activation of signaling pathways in these CAR-T cells when co-cultured with CD19+ and CD19-knockout NALM6 cells. These findings revealed that CD19-CAR-T cells stemmed from iPSCs demonstrated ERK1/2 phosphorylation at Thr202/Thr204 and PLCγ phosphorylation at Ser1248, mirroring the pattern observed in traditional CD19-targeting CAR-T cells. Notably, this phosphorylation was specifically dependent on the existence of CD19 antigen [50].
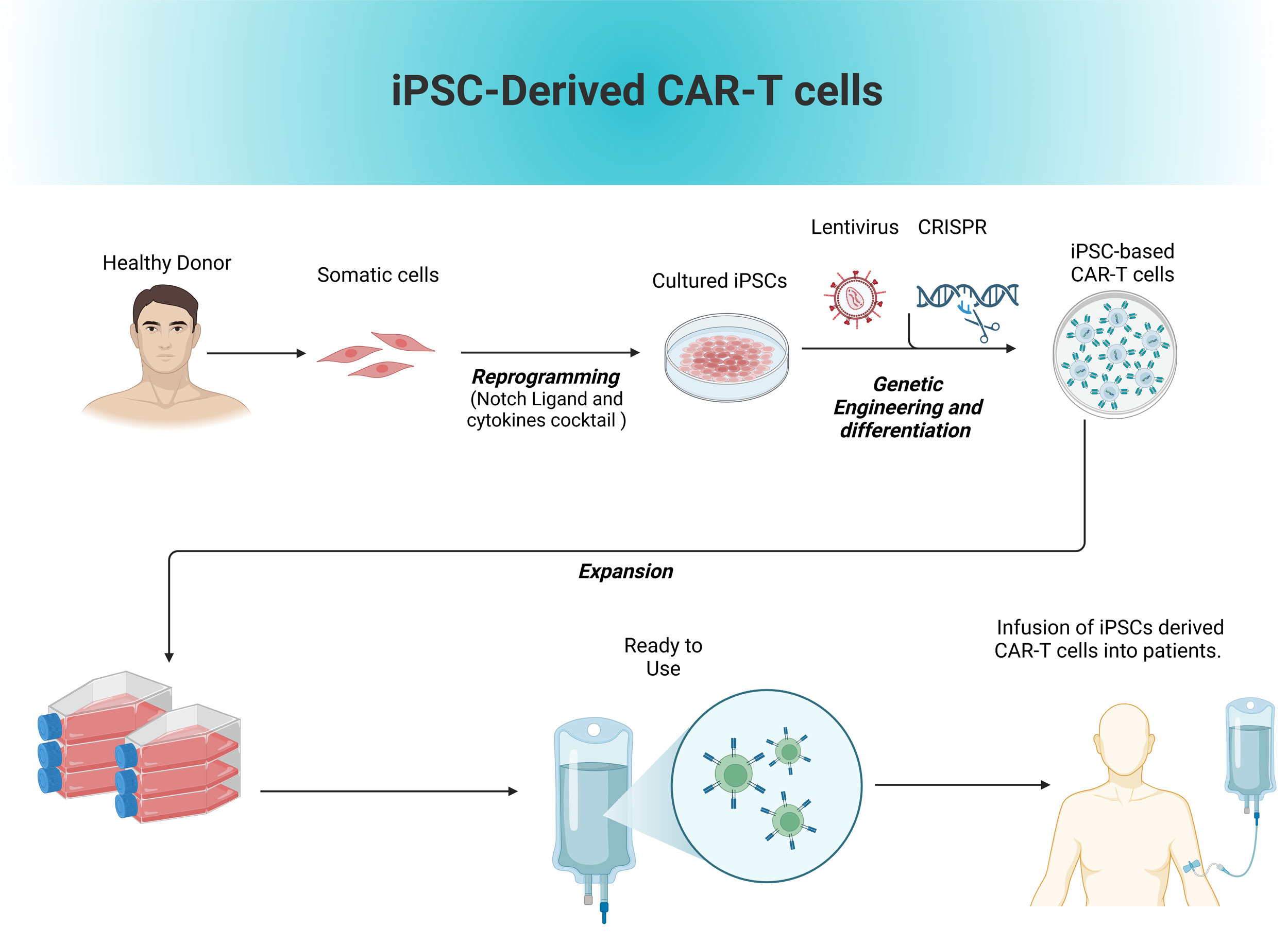
Current approaches to reduce EZH1 enhancer activity during T-cell differentiation primarily rely on genome-integrating viral vectors. Although dual EZH1/EZH2 inhibitors have shown promise in promoting NK cell development, small-molecule treatments have not been able to replicate the effects of shRNA-mediated EZH1 knockdown on iPSC-derived T cell differentiation. Considering the absence of EZH1-specific inhibitors that do not block EZH2, it is crucial to develop non-integrating gene knockdown methods to bring EZH1-targeted T-cell therapies into clinical use [57]. Furthermore, genetic modification strategies, such as incorporating immune-boosting genes (like IL-15, IL-2, IL-7, and IL-18), warrant investigation [58]. Exploring alternative cell types, including NK and iNKT cells, may be worthwhile because of their reduced potential to cause GvHD and other adverse effects. Generating T cells and CAR-T cells outside the thymus, utilizing either monolayer or three-dimensional organoid co-culture techniques, continues to be a challenging endeavor. iPSC CAR-T cells produced using a monolayer co-culture approach displayed characteristics distinct of innate immune cells. Showed lower efficiency in antigen-specific cytotoxicity and cytokine production relative to traditional CAR-T cells [59]. Moreover, establishing a system that eliminates the need for mouse-derived feeder cells while supporting efficient T-cell differentiation and production is essential for clinical and translational applications. This advancement not only augments the safeness and scalability of T cell-based therapies, but also opens doors to more effective treatments, advancing our progress towards realizing the absolute potency of immunotherapy in combating cancer and other illnesses [50].
(i) Research efforts should be directed towards optimized production methods with practical opportunities for scalability.
(ii) In production of CAR-T cells gene editing has a central role. Hence, it would be crucial to vigorously examine genotoxicity and predict long term consequences of gene editing.
(iii) Novel strategies should be developed that would prove invaluable for reducing the production time and treatment cost.
(iv) CAR-T therapies success is constrained in fighting the solid tumors, research in this direction would prove helpful in extending the CAR-T therapies arsenal to solid tumors.
(v) Long-term safety concerns should be rigorously investigated.
(vi) Research on streamlining approaches for data transferring and patenting guidelines related to the technology to reduce the time needed for regulatory reviews.
In the fight against cancer the potency of CAR-T therapies can be fully exploited by exploring novel approaches that can help in mitigating its limitations. The integration of iPSCs technology hold a transformative potential in revolutionizing the CAR-T treatment. iPSCs derived CAR-T cells would transform CAR therapies by enhancing its safety, effectiveness, and sustainability.
None.
Availability of data and materials
Data and materials are available on request from the authors.
Ethical policy
Not applicable.
Author contributions
TS contributed to draft, critical revision of the article, figure production and submitted the final version online.
Competing interests
I declare that there is no conflict of interest regarding the publication of this document.
Funding
None.
- Siegel RL, Giaquinto AN, Jemal A: Cancer statistics, 2024. CA Cancer J Clin 2024, 74(1): 12-49.
- YM A-W: Cancer Management: Overview. Handbook of Medical and Health Sciences in Developing Countries: Education, Practice, and Research Epub ahead of print.2024: 1-25.
- Joy R, Phair K, O'Hara R, Brady D: Recent advances and current challenges in CAR-T cell therapy. Biotechnol Lett 2024, 46(1): 115-126.
- Tao R, Han X, Bai X, Yu J, Ma Y, Chen W, Zhang D, Li Z: Revolutionizing cancer treatment: enhancing CAR-T cell therapy with CRISPR/Cas9 gene editing technology. Front Immunol 2024, 15: 1354825.
- Ong MZ, Kimberly SA, Lee WH, Ling M, Lee M, Tan KW, Foo JB, Yow HY, Sellappans R, Hamzah S: FDA-approved CAR T-cell Therapy: A Decade of Progress and Challenges. Curr Pharm Biotechnol 2024, 25(11): 1377-1393.
- Irizarry Gatell VM, Huang J, Puglianini OAC: CAR T-Cell Therapy. In: Anesth Oncol Surg. Epub ahead of print., edn.: Springer; 2024: 35-44.
- Elsallab M, Maus MV: Expanding access to CAR T cell therapies through local manufacturing. Nat Biotechnol 2023, 41(12): 1698-1708.
- Sainatham C, Yadav D, Dilli Babu A, Tallapalli JR, Kanagala SG, Filippov E, Murillo Chavez F, Ahmed N, Lutfi F: The current socioeconomic and regulatory landscape of immune effector cell therapies. Front Med (Lausanne) 2024, 11: 1462307.
- Wang L, Zhang L, Dunmall LC, Wang YY, Fan Z, Cheng Z, Wang Y: The dilemmas and possible solutions for CAR-T cell therapy application in solid tumors. Cancer Lett 2024, 591: 216871.
- Elmarasi M, Elkonaissi I, Elsabagh AA, Elsayed E, Elsayed A, Elsayed B, Elmakaty I, Yassin M: CAR-T cell therapy: Efficacy in management of cancers, adverse effects, dose-limiting toxicities and long-term follow up. Int Immunopharmacol 2024, 135: 112312.
- Mansoori S, Noei A, Maali A, Seyed-Motahari SS, Sharifzadeh Z: Recent updates on allogeneic CAR-T cells in hematological malignancies. Cancer Cell Int 2024, 24(1): 304.
- Mitra A, Barua A, Huang L, Ganguly S, Feng Q, He B: From bench to bedside: the history and progress of CAR T cell therapy. Front Immunol 2023, 14: 1188049.
- Chen YJ, Abila B, Mostafa Kamel Y: CAR-T: What Is Next? Cancers (Basel) 2023, 15(3): 663.
- Alidadi M, Barzgar H, Zaman M, Paevskaya OA, Metanat Y, Khodabandehloo E, Moradi V: Combining the induced pluripotent stem cell (iPSC) technology with chimeric antigen receptor (CAR)-based immunotherapy: recent advances, challenges, and future prospects. Front Cell Dev Biol 2024, 12: 1491282.
- Netsrithong R, Garcia-Perez L, Themeli M: Engineered T cells from induced pluripotent stem cells: from research towards clinical implementation. Front Immunol 2023, 14: 1325209.
- Jing R, Scarfo I, Najia MA, Lummertz da Rocha E, Han A, Sanborn M, Bingham T, Kubaczka C, Jha DK, Falchetti M, et al: EZH1 repression generates mature iPSC-derived CAR T cells with enhanced antitumor activity. Cell Stem Cell 2022, 29(8): 1181-1196.e6.
- Lahimchi MR, Maroufi F, Maali A: Induced Pluripotent Stem Cell-Derived Chimeric Antigen Receptor T Cells: The Intersection of Stem Cells and Immunotherapy. Cell Reprogram 2023, 25(5): 195-211.
- Nelson R: FDA investigating safety risks in CAR T-cell recipients. Lancet 2023, 402(10418): 2181.
- Yang J, He J, Zhang X, Li J, Wang Z, Zhang Y, Qiu L, Wu Q, Sun Z, Ye X, et al: Next-day manufacture of a novel anti-CD19 CAR-T therapy for B-cell acute lymphoblastic leukemia: first-in-human clinical study. Blood Cancer J 2022, 12(7): 104.
- Mishra AK, Gupta A, Dagar G, Das D, Chakraborty A, Haque S, Prasad CP, Singh A, Bhat AA, Macha MA, et al: CAR-T-Cell Therapy in Multiple Myeloma: B-Cell Maturation Antigen (BCMA) and Beyond. Vaccines (Basel) 2023, 11(11): 1721.
- Wu G, Guo S, Luo Q, Wang X, Deng W, Ouyang G, Pu JJ, Lei W, Qian W: Preclinical evaluation of CD70-specific CAR T cells targeting acute myeloid leukemia. Front Immunol 2023, 14: 1093750.
- Agliardi G, Dias J, Rampotas A, Garcia J, Roddie C: Accelerating and optimising CAR T-cell manufacture to deliver better patient products. Lancet Haematol 2024, S2352-3026(24): 00273-4.
- Young RM, Engel NW, Uslu U, Wellhausen N, June CH: Next-Generation CAR T-cell Therapies. Cancer Discov 2022, 12(7): 1625-1633.
- Asmamaw Dejenie T, Tiruneh GMM, Dessie Terefe G, Tadele Admasu F, Wale Tesega W, Chekol Abebe E: Current updates on generations, approvals, and clinical trials of CAR T-cell therapy. Hum Vaccin Immunother 2022, 18(6): 2114254.
- Mullard A: FDA approves fourth CAR-T cell therapy. Nat Rev Drug Discov 2021, 20(3): 166.
- Depil S, Duchateau P, Grupp SA, Mufti G, Poirot L: 'Off-the-shelf' allogeneic CAR T cells: development and challenges. Nat Rev Drug Discov 2020, 19(3): 185-199.
- Qasim W: Allogeneic CAR T cell therapies for leukemia. Am J Hematol 2019, 94(S1): S50-s54.
- Madison BB, Patil D, Richter M, Li X, Tong M, Cranert S, Wang X, Martin R, Xi H, Tan Y, et al: Cas-CLOVER is a novel high-fidelity nuclease for safe and robust generation of T(SCM)-enriched allogeneic CAR-T cells. Mol Ther Nucleic Acids 2022, 29: 979-995.
- Sidaway P: Allogeneic CAR T cells show promise. Nat Rev Clin Oncol 2022, 19(12): 748.
- Aftab BT, Sasu B, Krishnamurthy J, Gschweng E, Alcazer V, Depil S: Toward “off‐the‐shelf” allogeneic CAR T cells. Advances in Cell and Gene Therapy 2020, 3(3): e86.
- Sasu BJ, Lauron EJ, Schulz T, Cheng H-Y, Sommer C: Allogeneic CAR T Cell Therapy for Cancer. Annu Rev Cancer Biol 2023, 8: 227-243.
- Yan L GS, Wang X, Zhou X, Limsakul P, Wu Y: Boosting CAR-T cell therapy with CRISPR technology. hLife 2024, 2(8): 380-396.
- Hiltensperger M, Krackhardt AM: Current and future concepts for the generation and application of genetically engineered CAR-T and TCR-T cells. Front Immunol 2023, 14: 1121030.
- Stenger D, Stief TA, Kaeuferle T, Willier S, Rataj F, Schober K, Vick B, Lotfi R, Wagner B, Grünewald TGP, et al: Endogenous TCR promotes in vivo persistence of CD19-CAR-T cells compared to a CRISPR/Cas9-mediated TCR knockout CAR. Blood 2020, 136(12): 1407-1418.
- Cichocki F, van der Stegen SJC, Miller JS: Engineered and banked iPSCs for advanced NK- and T-cell immunotherapies. Blood 2023, 141(8): 846-855.
- Li W, Zhu X, Xu Y, Chen J, Zhang H, Yang Z, Qi Y, Hong J, Li Y, Wang G, et al: Simultaneous editing of TCR, HLA-I/II and HLA-E resulted in enhanced universal CAR-T resistance to allo-rejection. Front Immunol 2022, 13: 1052717.
- Mazinani M, Rahbarizadeh F: New cell sources for CAR-based immunotherapy. Biomark Res 2023, 11(1): 49.
- Glaser V, Flugel C, Kath J, Du W, Drosdek V, Franke C, Stein M, Pruß A, Schmueck-Henneresse M, Volk HD, et al: Combining different CRISPR nucleases for simultaneous knock-in and base editing prevents translocations in multiplex-edited CAR T cells. Genome Biol 2023, 24(1): 89.
- Abreu TR, Fonseca NA, Gonçalves N, Moreira JN: Current challenges and emerging opportunities of CAR-T cell therapies. J Control Release 2020, 319: 246-261.
- Huang J, Huang X, Huang J: CAR-T cell therapy for hematological malignancies: Limitations and optimization strategies. Front Immunol 2022, 13: 1019115.
- Zhang X, Zhang H, Lan H, Wu J, Xiao Y: CAR-T cell therapy in multiple myeloma: Current limitations and potential strategies. Front Immunol 2023, 14: 1101495.
- Abbasi M, Riaz, A., Khawar, M., Farooq, A., Majid, A., & Sheikh, N.: CAR-T-Cell Therapy: Present Progress and Future strategies. Biomed Res Ther 2022, 9(2): 4920-4929.
- Lundh S, Maji S, Melenhorst JJ: Next-generation CAR T cells to overcome current drawbacks. Int J Hematol 2021, 114(5): 532-543.
- Paul S, Lal G: Regulatory and effector functions of gamma-delta (γδ) T cells and their therapeutic potential in adoptive cellular therapy for cancer. Int J Cancer 2016, 139(5): 976-985.
- Li Y, Li G, Zhang J, Wu X, Chen X: The Dual Roles of Human γδ T Cells: Anti-Tumor or Tumor-Promoting. Front Immunol 2020, 11: 619954.
- Themeli M, Kloss CC, Ciriello G, Fedorov VD, Perna F, Gonen M, Sadelain M: Generation of tumor-targeted human T lymphocytes from induced pluripotent stem cells for cancer therapy. Nat Biotechnol 2013, 31(10): 928-933.
- Hidalgo I, Herrera-Merchan A, Ligos JM, Carramolino L, Nuñez J, Martinez F, Dominguez O, Torres M, Gonzalez S: Ezh1 is required for hematopoietic stem cell maintenance and prevents senescence-like cell cycle arrest. Cell Stem Cell 2012, 11(5): 649-662.
- Lavaert M, Bhandoola A: T cell development made EZ. Cell Stem Cell 2022, 29(8): 1155-1156.
- Fang M, Allen A, Luo C, Finn JD: Unlocking the potential of iPSC-derived immune cells: engineering iNK and iT cells for cutting-edge immunotherapy. Front Immunol 2024, 15: 1457629.
- Wang Z, McWilliams-Koeppen HP, Reza H, Ostberg JR, Chen W, Wang X, Huynh C, Vyas V, Chang WC, Starr R, et al: 3D-organoid culture supports differentiation of human CAR(+) iPSCs into highly functional CAR T cells. Cell Stem Cell 2022, 29(4): 515-527.e518.
- Seet CS, He C, Bethune MT, Li S, Chick B, Gschweng EH, Zhu Y, Kim K, Kohn DB, Baltimore D, et al: Generation of mature T cells from human hematopoietic stem and progenitor cells in artificial thymic organoids. Nat Methods 2017, 14(5): 521-530.
- Sharma A, Sances S, Workman MJ, Svendsen CN: Multi-lineage Human iPSC-Derived Platforms for Disease Modeling and Drug Discovery. Cell Stem Cell 2020, 26(3): 309-329.
- Wang X, Wu X, Tan B, Zhu L, Zhang Y, Lin L, Xiao Y, Sun A, Wan X, Liu S, et al: Allogeneic CD19-targeted CAR-T therapy in patients with severe myositis and systemic sclerosis. Cell 2024, 187(18): 4890-4904.e4899.
- Silvestre RN, Eitler J, de Azevedo JTC, Tirapelle MC, Fantacini DMC, de Souza LEB, Swiech K, Covas DT, Calado RT, Montero PO, et al: Engineering NK-CAR.19 cells with the IL-15/IL-15Rα complex improved proliferation and anti-tumor effect in vivo. Front Immunol 2023, 14: 1226518.
- Zhou Y, Husman T, Cen X, Tsao T, Brown J, Bajpai A, Li M, Zhou K, Yang L: Interleukin 15 in Cell-Based Cancer Immunotherapy. Int J Mol Sci 2022, 23(13): 7311.
- Zhou Y, Farooq MA, Ajmal I, He C, Gao Y, Guo D, Duan Y, Jiang W: Co-expression of IL-4/IL-15-based inverted cytokine receptor in CAR-T cells overcomes IL-4 signaling in immunosuppressive pancreatic tumor microenvironment. Biomed Pharmacother 2023, 168: 115740.
- Kang N, Eccleston M, Clermont PL, Latarani M, Male DK, Wang Y, Crea F: EZH2 inhibition: a promising strategy to prevent cancer immune editing. Epigenomics 2020, 12(16): 1457-1476.
- Ye J, Liu Q, He Y, Song Z, Lin B, Hu Z, Hu J, Ning Y, Cai C, Li Y: Combined therapy of CAR-IL-15/IL-15Rα-T cells and GLIPR1 knockdown in cancer cells enhanced anti-tumor effect against gastric cancer. J Transl Med 2024, 22(1): 171.
- Zhou Y, Li M, Zhou K, Brown J, Tsao T, Cen X, Husman T, Bajpai A, Dunn ZS, Yang L: Engineering Induced Pluripotent Stem Cells for Cancer Immunotherapy. Cancers (Basel) 2022, 14(9): 2266.
Asia-Pacific Journal of Oncology
print ISSN: 2708-7980, online ISSN: 2708-7999
Copyright © Asia Pac J Oncol. This work is licensed under a Creative Commons Attribution-NonCommercial-No Derivatives 4.0 International (CC BY-NC-ND 4.0) License.