Review | Open Access
Review of immunotherapy in non-small cell lung cancer: mechanisms, clinical applications, and future prospects
Palwasha Habib1
1Department of medicine, Mohtarma Benazir Bhutto Shaheed medical college, Mirpur, Azad Jammu and Kashmir (AJK).
Correspondence: Palwasha Habib (Department of medicine, Mohtarma Benazir Bhutto Shaheed medical college, Mirpur, AJK; Email: drpalwashahabib786@gmail.com).
Asia-Pacific Journal of Oncology 2024, 5: 39-45. https://doi.org/10.32948/ajo.2024.09.08
Received: 15 Aug 2024 | Accepted: 08 Sep 2024 | Published online: 11 Sep 2024
Key words non-small cell lung cancer (NSCLC), PD-1/PD-L1 pathway, CTLA-4, oncolytic virus, immunotherapy
PD-1/PD-L1 pathway
The PD-1/PD-L1 pathway is a crucial regulatory mechanism in the immune system, primarily involving the programmed cell death protein 1 (PD-1) receptor and its ligand, programmed death-ligand 1 (PD-L1). These components play crucial roles in immune regulation. PD-1 is a receptor found on the surface of activated T cells, B cells, and other immune cells [7]. It interacts with PD-L1, a ligand expressed in various cell types, including tumor cells and antigen-presenting cells such as dendritic cells and macrophages [8]. This pathway is essential for maintaining immune system balance and preventing autoimmune responses. However, in tumor microenvironment, this mechanism is often exploited to evade immune surveillance [9]. In NSCLC, the PD-1/PD-L1 pathway plays a crucial role in the mechanism of immune evasion. Tumor cells can upregulate PD-L1 expression, which binds to PD-1 on T cells, inhibiting their activity and facilitating immune surveillance [10]. Targeting this pathway provides a promising strategy for NSCLC treatment. PD-1/PD-L1 inhibitors disrupt the interaction between PD-1 and PD-L1, reactivating T-cell function and enhancing the immune system's ability to recognize and eliminate tumor cells. Specifically, PD-1 inhibitors like Nivolumab and Pembrolizumab bind to the PD-1 receptor, blocking its interaction with PD-L1, which in turn lifts the suppression of T-cells. Similarly, PD-L1 inhibitors like Atezolizumab bind to PD-L1, preventing its interaction with PD-1 and also releasing T-cell inhibition.
CTLA-4 pathway
The CTLA-4 pathway is another crucial immune checkpoint that regulates immune system activity and prevents autoimmune reactions involving CTLA-4 and B7 molecules [11]. In NSCLC, tumor cells promote CTLA-4 expression or enhance B7 activity, thereby inhibiting T cell function and facilitating immune evasion. The activation of the CTLA-4 pathway prevents T cells from exerting their antitumor effects, promoting tumor growth and spread [12]. CTLA-4 inhibitors block the interaction between CTLA-4 and B7 molecules, releasing the inhibition on T cells, thereby restoring and enhancing their antitumor activity. Specifically, CTLA-4 inhibitors like Ipilimumab bind to CTLA-4, preventing its interaction with B7 molecules and enhancing T cell activation and proliferation [13]. CTLA-4 inhibitors increase the activity of antigen-presenting cells, leading to the presentation of more tumor antigens to T-cells and enhancing the immune response.
Oncolytic viruses
Oncolytic viruses, as a novel immunotherapy mechanism, use naturally occurring or genetically engineered viruses to selectively infect and kill tumor cells [14]. These viruses directly destroy tumor cells and activate the host immune system to combat the tumor [15]. NSCLC cells express specific receptors targeted by oncolytic viruses. For instance, certain adenoviruses selectively infect NSCLC cells by binding to the Coxsackievirus and Adenovirus Receptor (CAR) present on their surfaces [16]. NSCLC cells typically have defective antiviral defence mechanisms, which facilitate the replication and dissemination of oncolytic viruses. Oncolytic viruses, upon entering NSCLC cells, proliferate and release new viral particles, and disrupting the cell membrane, leading to cell lysis and death. Besides directly killing NSCLC cells, oncolytic viruses can also stimulate the host immune system's antitumor response [17], [18].
Chimeric antigen receptor T-cells (CAR-T cells)
Chimeric Antigen Receptor T-cell Therapy is an advanced immunotherapy that genetically modifies a patient's T-cells to express chimeric antigen receptors (CARs), which can specifically recognize and kill tumor cells. This therapy has achieved significant success in hematological malignancies and is being explored for its application in solid tumors, including NSCLC [19], [20]. The procedure begins with the extraction and isolation of T-cells from the patient's peripheral blood. Subsequently, it introduces a gene that encodes a single-chain antibody (scFv) capable of recognizing tumor-specific antigens, along with a chimeric antigen receptor (CAR) that activates the T-cell signaling domain. This gene is delivered into the T cells using viral vectors, typically lentiviruses or retroviruses [21]. The genetically modified CAR-T cells undergo in vitro expansion before being reintroduced into the patient via intravenous injection. Once administered, the cells recognize and bind to antigens on the surface of tumor cells through their CAR structure specificity in vivo. Following binding to tumor cells, the CAR-T cells are activated and release cytotoxic particles (such as perforin and granzyme), killing tumor cells [22].
Overall, traditional immunotherapy (PD-1/PD-L1 inhibitors) has broad applicability and durability. However, it also has limited response rates, drug resistance, and side effects. Such therapies have established themselves as critical components of cancer treatment, offering a valuable foundation for developing novel immunotherapeutic approaches. New immunotherapy is characterized by high specificity and efficiency, but may face challenges such as immune system interference, safety, and limited efficacy. These therapies may show greater potential in personalized treatment and specific cancer types.
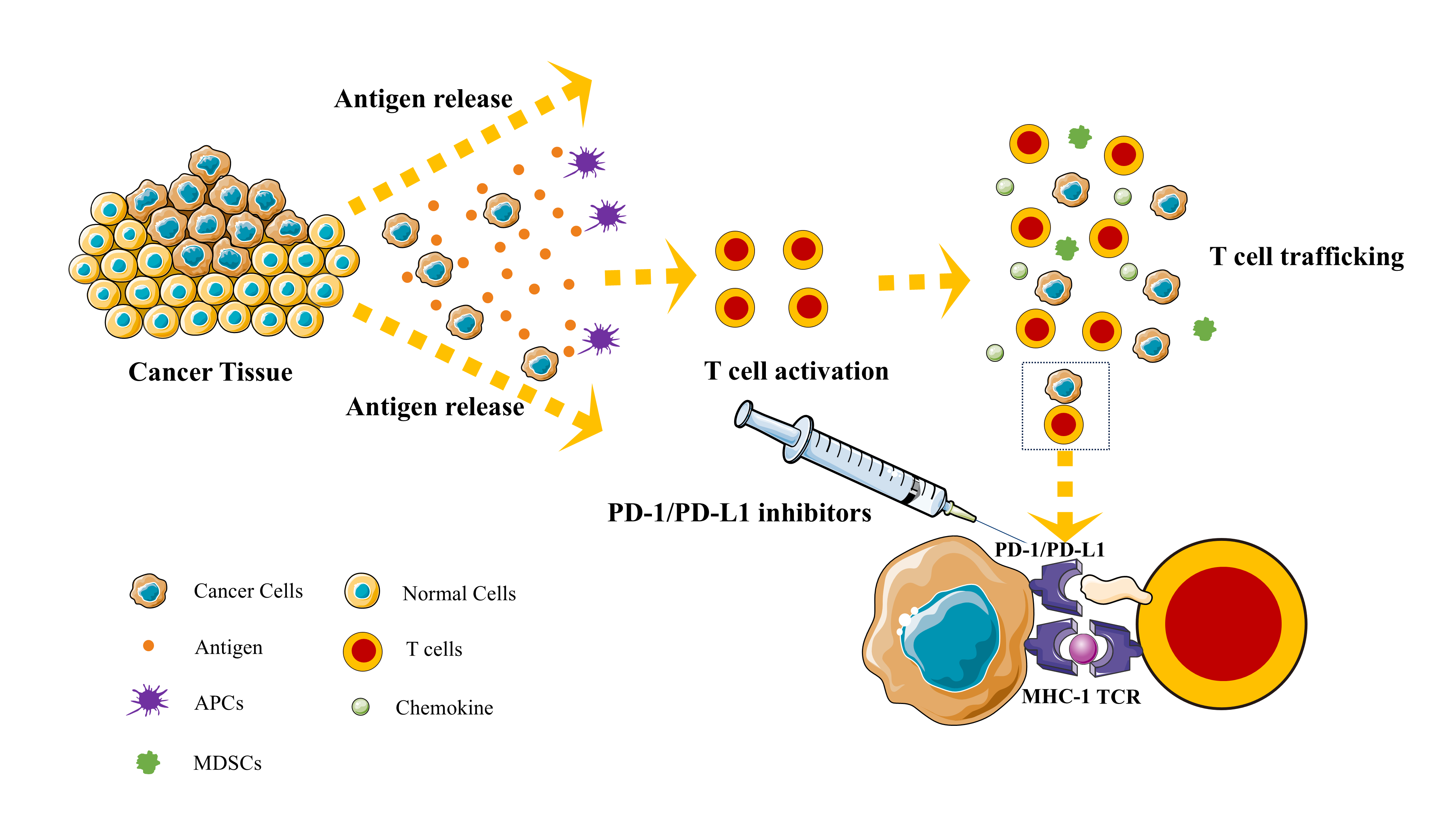
Nivolumab (Opdivo)
Nivolumab (Opdivo) is the first PD-1 inhibitor approved for the treatment of NSCLC. It is involved in two key clinical trials, CheckMate-017 and CheckMate-057, which compared the efficacy of Nivolumab with docetaxel in patients with advanced squamous NSCLC [23], [24]. The findings revealed a median overall survival (OS) of 9.2 months for the Nivolumab group, surpassing the 6.0 months recorded for the docetaxel group. The 1-year survival rate in the Nivolumab group was 42%, significantly higher than the 24% observed in the docetaxel group. These clinical trials highlight the substantial progress Nivolumab has achieved in the treatment of NSCLC, notably extending both the overall survival (OS) and progression-free survival (PFS) of patients with advanced NSCLC.
New immune checkpoints
The remarkable success of PD-1/PD-L1 and CTLA-4 inhibitors in cancer therapy enables researchers to explore novel immune checkpoints to enhance the efficacy and overcome the limitations of current treatments. LAG-3 (lymphocyte activation gene 3), a receptor found on activated T cells, natural killer (NK) cells, and some B cells, typically inhibits T cell function through its interaction with MHC class II molecules. Studies indicate that the combination of LAG-3 inhibitors and PD-1 inhibitors can overcome resistance to PD-1 monotherapy and improve efficacy [25]. TIM-3 (T-cell immunoglobulin and mucin 3) is expressed on T-cells, NK cells, and monocytes as a negative regulatory molecule that inhibits T-cell function when it binds to its ligands (such as Galectin-9). This interaction can induce immune suppression signals [26]. TIM-3 inhibitors may release T-cell inhibition through this mechanism and enhance the immune system's antitumor ability, especially in PD-1-resistant patients [27]. TIGIT (T-cell immunoglobulin and ITIM domain) is expressed on T cells and NK cells, binds to CD155, transmits inhibitory signals, reduces the activity of T cells and NK cells, and can also compete with CD226 to bind to CD155, thereby inhibiting immune activation [28]. Preliminary studies have shown that in some patients, the combination of TIGIT inhibitors and PD-L1 inhibitors (such as Atezolizumab) exhibits enhanced antitumor activity. B7-H3 (CD276), expressed on multiple cell types, including tumor cells, participates in immune suppression and plays a role in tumor immune escape [29]. VISTA (V-domain Ig suppressor of T cell activation) is a negative immune regulatory molecule expressed on myeloid cells and some T cells [30]. It suppresses T cell activation and proliferation by binding to its ligands. As a new immune checkpoint, VISTA inhibitors are undergoing early clinical trials to explore their potential in cancer treatment [31], [32]. Research on new immune checkpoints is rapidly developing, with the aim of overcoming the limitations of existing immunotherapy and providing patients with more treatment options [33]. An in-depth understanding of these checkpoints underlying mechanisms and their roles in the tumor microenvironment might enable researchers to develop new inhibitors and combination therapies to enhance the therapeutic efficacy of NSCLC and other cancers.
Oncolytic viruses
Oncolytic viruses have primarily developed four strains: Oncorine (H101) [34], Talimogene laherparepvec (T-VEC) [35], Reovirus [36], and measles virus [37]. H101 is a genetically modified adenovirus that was first approved in China for the treatment of head and neck squamous cell carcinoma. Its research in NSCLC has shown potential, especially when used in conjunction with chemotherapy, to enhance treatment efficacy. T-VEC is a modified herpes simplex virus (HSV) approved for unresectable melanoma and is under investigation for its efficacy in NSCLC [38]. Early clinical trials indicate that T-VEC induces an immune response in NSCLC patients and exhibits a synergistic effect when combined with PD-1 inhibitors. Reovirus, a naturally occurring virus, selectively infect and kill tumor cells. Clinical trials in NSCLC indicate that Reovirus has good safety and potential efficacy, particularly when combined with immune checkpoint inhibitors. The genetically engineered measles virus is used to treat various cancers, including NSCLC. Preclinical studies show that the measles virus can selectively infect and kill NSCLC cells, causing a strong antitumor immune response.
Biomarkers
Identifying and applying biomarkers significantly enhances treatment response prediction, optimizes treatment plans, and improves efficacy in immunotherapy in NSCLC. Tumor mutation burden (TMB) is the number of mutations present in the tumor genome per million base pairs (Mb). High TMB is often associated with the generation of new antigens, which can more effectively stimulate immune responses. Therefore, TMB is considered an important biomarker for predicting immune therapy responses [39]. In the CheckMate-227 clinical trial, the efficacy of Nivolumab (PD-1 inhibitor) in combination with Ipilimumab (CTLA-4 inhibitor) was evaluated in patients with high TMB NSCLC. The results indicated that patients with high TMB (≥ 10 mutations/Mb) experienced significantly longer progression-free survival (PFS) and overall survival (OS), underscoring the association of high TMB with improved therapeutic outcomes [40]. Therefore, TMB testing can be an important criterion for screening suitable immunotherapy patients.
Circulating tumor DNA (ctDNA) emerges as a pivotal biomarker in NSCLC immunotherapy, consisting of free DNA fragments released from tumor cells into the bloodstream. ctDNA has demonstrated significant potential in cancer detection and monitoring, particularly in NSCLC immunotherapy, where it offers dynamic, non-invasive insights into tumor characteristics and treatment response [41]. Currently, the primary methods for detecting ctDNA include digital PCR (dPCR), next-generation sequencing (NGS), and BEAMing technology. The ctDNA has been shown to decrease after immunotherapy treatment, indicating a more favorable treatment response and serving as a predictor of treatment outcomes. Furthermore, regular monitoring of ctDNA levels allows for real-time assessment of immunotherapy efficacy. An increase in ctDNA levels may indicate disease progression or recurrence, and early intervention can improve patient prognosis [42]. Analysis of ctDNA also facilitates the identification of new drug-resistant mutations, enabling timely adjustments to treatment plans. Compared with traditional tissue biopsies, ctDNA testing can be performed through simple blood extraction, reducing the pain and risk for patients. ctDNA can reflect the heterogeneity of tumors throughout the body and provide more comprehensive genomic information than single-tissue biopsies [43]. Despite the continuous advancement of ctDNA detection technology, there is still a need to improve its sensitivity and specificity further, especially in low-frequency mutation detection. Standardized detection processes and analytical methods need to be established to ensure comparability of results between different laboratories. Consequently, extensive clinical studies are imperative to confirm the utility of ctDNA in predicting immunotherapy responses and monitoring for drug resistance.
Neoantigen, an antigen produced by unique mutations in tumor cells that are not expressed in normal cells, can be detected by the immune system, leading to a robust antitumor response. Neoantigen load refers to the number of neoantigens produced in tumor cells, and a higher neoantigen load is usually associated with better immune therapy response [44]. Its mechanism of action involves the presentation on T-cells through major histocompatibility complex (MHC) molecules, triggering T-cells to recognize and attack tumor cells. Neoantigen increases the probability of the immune system recognizing tumors, thereby enhancing immune surveillance function. The KEYNOTE-010 clinical trial evaluated the efficacy of Pembrolizumab (PD-1 inhibitor) in PD-L1 positive NSCLC patients, indicating a positive correlation between neoantigen burden and Pembrolizumab treatment response. Specifically, patients with high antigen burdens are showing better treatment outcomes [45], highlighting the potential of neoantigen burden to be used as a biomarker for selecting suitable immunotherapy patients. Patients with a high antigen burden are more likely to benefit from immune checkpoint inhibitor therapy. In the future, it can be combined with other biomarkers such as TMB, PD-L1 expression, and TILs to improve prediction accuracy.
Table 1. Characteristics of immune checkpoint inhibitors in the treatment of NSCLC. |
||||
Drug/Therapy |
Target |
Primary indication |
Combination therapy strategy |
Major efficacy |
Nivolumab (Opdivo) |
PD-1 inhibitor |
Advanced or metastatic NSCLC patients with progression after chemotherapy |
Combined with Ipilimumab |
Significantly prolongs OS in advanced NSCLC patients, good tolerability |
Pembrolizumab (Keytruda) |
PD-1 inhibitor |
Advanced or metastatic NSCLC patients with high PD-L1 expression, as a first-line therapy |
Combined with chemotherapy |
Significantly prolongs OS and PFS, especially in patients with PD-L1 expression ≥50% |
Atezolizumab (Tecentriq) |
PD-L1 inhibitor |
Advanced or metastatic NSCLC patients with progression after chemotherapy |
Combined with chemotherapy and bevacizumab |
Significantly prolongs OS, especially in non-squamous cell carcinoma patients |
Ipilimumab (Yervoy) |
CTLA-4 inhibitor |
Primarily for melanoma treatment, shows potential in NSCLC |
Combined with Nivolumab |
Combined therapy shows enhanced antitumor activity, especially in patients with high tumor mutation burden |
Despite its efficacy in NSCLC treatment, the side effects associated with immunotherapy require vigilant monitoring by clinicians and patients. Early detection, timely intervention, and individualized management are crucial to minimizing the adverse impact on patients' quality of life and optimizing the benefits of immunotherapy.
No applicable.
Availability of data and materials
Data and materials are available on request from the authors.
Ethical policy
Not applicable.
Author contributions
PH conceptualized, designed, conducted research, wrote the draft and approve the final manuscript.
Competing interests
The authors have no conflicts of interest regarding the publication of this paper.
Funding
None.
- Jonna S, Subramaniam DS: Molecular diagnostics and targeted therapies in non-small cell lung cancer (NSCLC): an update. Discov Med 2019, 27(148): 167-170.
- Chen Z, Fillmore CM, Hammerman PS, Kim CF, Wong KK: Non-small-cell lung cancers: a heterogeneous set of diseases. Nat Rev Cancer 2014, 14(8): 535-546.
- Passaro A, Mok TSK, Attili I, Wu YL, Tsuboi M, de Marinis F, Peters S: Adjuvant Treatments for Surgically Resected Non-Small Cell Lung Cancer Harboring EGFR Mutations: A Review. JAMA Oncol 2023, 9(8): 1124-1131.
- Rossi A, Di Maio M: Platinum-based chemotherapy in advanced non-small-cell lung cancer: optimal number of treatment cycles. Expert Rev Anticancer Ther 2016, 16(6): 653-660.
- Yu Y, Zeng D, Ou Q, Liu S, Li A, Chen Y, Lin D, Gao Q, Zhou H, Liao W et al: Association of Survival and Immune-Related Biomarkers With Immunotherapy in Patients With Non-Small Cell Lung Cancer: A Meta-analysis and Individual Patient-Level Analysis. JAMA Netw Open 2019, 2(7): e196879.
- Lahiri A, Maji A, Potdar PD, Singh N, Parikh P, Bisht B, Mukherjee A, Paul MK: Lung cancer immunotherapy: progress, pitfalls, and promises. Mol Cancer 2023, 22(1): 40.
- Ai L, Xu A, Xu J: Roles of PD-1/PD-L1 Pathway: Signaling, Cancer, and Beyond. Adv Exp Med Biol 2020, 1248: 33-59.
- Han Y, Liu D, Li L: PD-1/PD-L1 pathway: current researches in cancer. Am J Cancer Res 2020, 10(3): 727-742.
- Salmaninejad A, Valilou SF, Shabgah AG, Aslani S, Alimardani M, Pasdar A, Sahebkar A: PD-1/PD-L1 pathway: Basic biology and role in cancer immunotherapy. J Cell Physiol 2019, 234(10): 16824-16837.
- Xia L, Liu Y, Wang Y: PD-1/PD-L1 Blockade Therapy in Advanced Non-Small-Cell Lung Cancer: Current Status and Future Directions. Oncologist 2019, 24(Suppl 1): S31-s41.
- Rowshanravan B, Halliday N, Sansom DM: CTLA-4: a moving target in immunotherapy. Blood 2018, 131(1): 58-67.
- Hosseini A, Gharibi T, Marofi F, Babaloo Z, Baradaran B: CTLA-4: From mechanism to autoimmune therapy. Int Immunopharmacol 2020, 80: 106221.
- Naimi A, Mohammed RN, Raji A, Chupradit S, Yumashev AV, Suksatan W, Shalaby MN, Thangavelu L, Kamrava S, Shomali N et al: Tumor immunotherapies by immune checkpoint inhibitors (ICIs); the pros and cons. Cell Commun Signal 2022, 20(1): 44.
- Mondal M, Guo J, He P, Zhou D: Recent advances of oncolytic virus in cancer therapy. Hum Vaccin Immunother 2020, 16(10): 2389-2402.
- Malhotra J, Kim ES: Oncolytic Viruses and Cancer Immunotherapy. Curr Oncol Rep 2023, 25(1): 19-28.
- Niemann J, Kühnel F: Oncolytic viruses: adenoviruses. Virus Genes 2017, 53(5): 700-706.
- Volovat SR, Scripcariu DV, Vasilache IA, Stolniceanu CR, Volovat C, Augustin IG, Volovat CC, Ostafe MR, Andreea-Voichița SG, Bejusca-Vieriu T et al: Oncolytic Virotherapy: A New Paradigm in Cancer Immunotherapy. Int J Mol Sci 2024, 25(2): 1180.
- Ma R, Li Z, Chiocca EA, Caligiuri MA, Yu J: The emerging field of oncolytic virus-based cancer immunotherapy. Trends Cancer 2023, 9(2): 122-139.
- Ma S, Li X, Wang X, Cheng L, Li Z, Zhang C, Ye Z, Qian Q: Current Progress in CAR-T Cell Therapy for Solid Tumors. Int J Biol Sci 2019, 15(12): 2548-2560.
- Alcantara M, Du Rusquec P, Romano E: Current Clinical Evidence and Potential Solutions to Increase Benefit of CAR T-Cell Therapy for Patients with Solid Tumors. Oncoimmunology 2020, 9(1): 1777064.
- Martinez M, Moon EK: CAR T Cells for Solid Tumors: New Strategies for Finding, Infiltrating, and Surviving in the Tumor Microenvironment. Front Immunol 2019, 10: 128.
- Kosti P, Maher J, Arnold JN: Perspectives on Chimeric Antigen Receptor T-Cell Immunotherapy for Solid Tumors. Front Immunol 2018, 9: 1104.
- Borghaei H, Gettinger S, Vokes EE, Chow LQM, Burgio MA, de Castro Carpeno J, Pluzanski A, Arrieta O, Frontera OA, Chiari R et al: Five-Year Outcomes From the Randomized, Phase III Trials CheckMate 017 and 057: Nivolumab Versus Docetaxel in Previously Treated Non-Small-Cell Lung Cancer. J Clin Oncol 2021, 39(7): 723-733.
- Horn L, Spigel DR, Vokes EE, Holgado E, Ready N, Steins M, Poddubskaya E, Borghaei H, Felip E, Paz-Ares L et al: Nivolumab Versus Docetaxel in Previously Treated Patients With Advanced Non-Small-Cell Lung Cancer: Two-Year Outcomes From Two Randomized, Open-Label, Phase III Trials (CheckMate 017 and CheckMate 057). J Clin Oncol 2017, 35(35): 3924-3933.
- Aggarwal V, Workman CJ, Vignali DAA: LAG-3 as the third checkpoint inhibitor. Nat Immunol 2023, 24(9):1415-1422.
- Sauer N, Janicka N, Szlasa W, Skinderowicz B, Kołodzińska K, Dwernicka W, Oślizło M, Kulbacka J, Novickij V, Karłowicz-Bodalska K: TIM-3 as a promising target for cancer immunotherapy in a wide range of tumors. Cancer Immunol Immunother 2023, 72(11): 3405-3425.
- Gomes de Morais AL, Cerdá S, de Miguel M: New Checkpoint Inhibitors on the Road: Targeting TIM-3 in Solid Tumors. Curr Oncol Rep 2022, 24(5): 651-658.
- Harjunpää H, Guillerey C: TIGIT as an emerging immune checkpoint. Clin Exp Immunol 2020, 200(2): 108-119.
- Zhou WT, Jin WL: B7-H3/CD276: An Emerging Cancer Immunotherapy. Front Immunol 2021, 12: 701006.
- Yuan L, Tatineni J, Mahoney KM, Freeman GJ: VISTA: A Mediator of Quiescence and a Promising Target in Cancer Immunotherapy. Trends Immunol 2021, 42(3): 209-227.
- Wu C, Cao X, Zhang X: VISTA inhibitors in cancer immunotherapy: a short perspective on recent progresses. RSC Med Chem 2021, 12(10): 1672-1679.
- Shekari N, Shanehbandi D, Kazemi T, Zarredar H, Baradaran B, Jalali SA: VISTA and its ligands: the next generation of promising therapeutic targets in immunotherapy. Cancer Cell Int 2023, 23(1): 265.
- Kong X: Discovery of New Immune Checkpoints: Family Grows Up. Adv Exp Med Biol 2020, 1248: 61-82.
- Liang M: Oncorine, the World First Oncolytic Virus Medicine and its Update in China. Curr Cancer Drug Targets 2018, 18(2): 171-176.
- Salloum A, Koblinski J, Bazzi N, Zeitouni NC: Talimogene Laherparepvec in Non-Melanoma Cancers. J Clin Aesthet Dermatol 2021, 14(11): 18-25.
- Kumar V, Giacomantonio MA, Gujar S: Role of Myeloid Cells in Oncolytic Reovirus-Based Cancer Therapy. Viruses 2021, 13(4): 654.
- Blechacz B, Russell SJ: Measles virus as an oncolytic vector platform. Curr Gene Ther 2008, 8(3): 162-175.
- Shalhout SZ, Miller DM, Emerick KS, Kaufman HL: Therapy with oncolytic viruses: progress and challenges. Nat Rev Clin Oncol 2023, 20(3): 160-177.
- Jardim DL, Goodman A, de Melo Gagliato D, Kurzrock R: The Challenges of Tumor Mutational Burden as an Immunotherapy Biomarker. Cancer Cell 2021, 39(2): 154-173.
- Paz-Ares LG, Ramalingam SS, Ciuleanu TE, Lee JS, Urban L, Caro RB, Park K, Sakai H, Ohe Y, Nishio M et al: First-Line Nivolumab Plus Ipilimumab in Advanced NSCLC: 4-Year Outcomes From the Randomized, Open-Label, Phase 3 CheckMate 227 Part 1 Trial. J Thorac Oncol 2022, 17(2): 289-308.
- Pessoa LS, Heringer M, Ferrer VP: ctDNA as a cancer biomarker: A broad overview. Crit Rev Oncol Hematol 2020, 155: 103109.
- Stadler JC, Belloum Y, Deitert B, Sementsov M, Heidrich I, Gebhardt C, Keller L, Pantel K: Current and Future Clinical Applications of ctDNA in Immuno-Oncology. Cancer Res 2022, 82(3): 349-358.
- Snyder A, Morrissey MP, Hellmann MD: Use of Circulating Tumor DNA for Cancer Immunotherapy. Clin Cancer Res 2019, 25(23): 6909-6915.
- Peng M, Mo Y, Wang Y, Wu P, Zhang Y, Xiong F, Guo C, Wu X, Li Y, Li X et al: Neoantigen vaccine: an emerging tumor immunotherapy. Mol Cancer 2019, 18(1): 128.
- Herbst RS, Garon EB, Kim DW, Cho BC, Gervais R, Perez-Gracia JL, Han JY, Majem M, Forster MD, Monnet I et al: Five Year Survival Update From KEYNOTE-010: Pembrolizumab Versus Docetaxel for Previously Treated, Programmed Death-Ligand 1-Positive Advanced NSCLC. J Thorac Oncol 2021, 16(10): 1718-1732.
- Tan S, Li D, Zhu X: Cancer immunotherapy: Pros, cons and beyond. Biomed Pharmacother 2020, 124: 109821.
- Ke W, Zhang L, Dai Y: The role of IL-6 in immunotherapy of non-small cell lung cancer (NSCLC) with immune-related adverse events (irAEs). Thorac Cancer 2020, 11(4): 835-839.
- Santini FC, Rizvi H, Plodkowski AJ, Ni A, Lacouture ME, Gambarin-Gelwan M, Wilkins O, Panora E, Halpenny DF, Long NM et al: Safety and Efficacy of Re-treating with Immunotherapy after Immune-Related Adverse Events in Patients with NSCLC. Cancer Immunol Res 2018, 6(9): 1093-1099.
- Mamdani H, Matosevic S, Khalid AB, Durm G, Jalal SI: Immunotherapy in Lung Cancer: Current Landscape and Future Directions. Front Immunol 2022, 13: 823618.
- Curran MA, Montalvo W, Yagita H, Allison JP: PD-1 and CTLA-4 combination blockade expands infiltrating T cells and reduces regulatory T and myeloid cells within B16 melanoma tumors. Proc Natl Acad Sci U S A 2010, 107(9): 4275-4280.
- Dao J, Conway PJ, Subramani B, Meyyappan D, Russell S, Mahadevan D: Using cfDNA and ctDNA as Oncologic Markers: A Path to Clinical Validation. Int J Mol Sci 2023, 24(17): 13219.
Asia-Pacific Journal of Oncology
print ISSN: 2708-7980, online ISSN: 2708-7999
Copyright © Asia Pac J Oncol. This work is licensed under a Creative Commons Attribution-NonCommercial-No Derivatives 4.0 International (CC BY-NC-ND 4.0) License.